Among Earth’s scintillating biodiversity, Cyanobacteria hardly appear remarkable. Yet, despite their size, these bacteria have played a crucial role in the evolution of the Earth as we know it.
Cyanobacteria (Cyan: Blue-green) are a group of photosynthetic microorganisms, that can be found in fresh and brackish water and range from 0.5 to 100 micrometers in size. The shape of Cyanobacteria can range from spheroidal, rod-shaped, filamentous or tubular and move by secreting slime which facilitates their gliding movements across surfaces.
Cyanobacteria were the first organisms on earth to develop the capability to photosynthesize. By fixing nitrogen and releasing oxygen, these bacteria are responsible for the formation of Earth’s oxygen rich atmosphere. In this article, let us explore the characteristics of these microorganisms and the vital role they play in our ecosystem.
Evolution of Cyanobacteria
Scientists learn about the evolution and origin of cyanobacteria by studying their fossil records. Microorganisms living in shallow water habitats tend to trap sediments leading to their solidification. These fossil formations called stromatolites are some of the oldest records of ancient life on earth.
Cyanobacteria fossils are fairly easy to recognize using SEM/TEM due to their unique morphology. Furthermore, the chemical residues and breakdown products left behind in the rocks were used to elucidate their early metabolism. The genetic sequences of cyanobacteria obtained from fossils have been extensively analyzed and accumulated in public databases.
Scientists use these databases to carry out phylogenetic reconstructions of modern organisms. However, one must always remember that these reconstruction processes do tend to have limitations and biases.
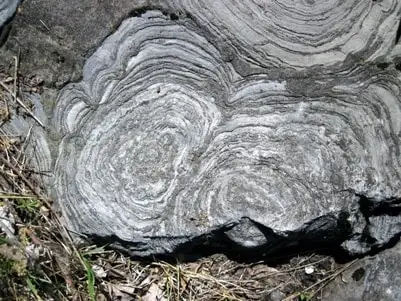
The general consensus among scientists is that cyanobacteria originated about 2.4 billion years ago. However, the oldest cyanobacteria fossil recovered from Western Australia is dated over 3.5 billion years old. At this point in time, Earth’s atmosphere was highly reducing, with oxygen concentrations remaining below 1%. When cyanobacteria gained the ability to carry out oxygenic photosynthesis, the oxygen concentration in the atmosphere rose rapidly.
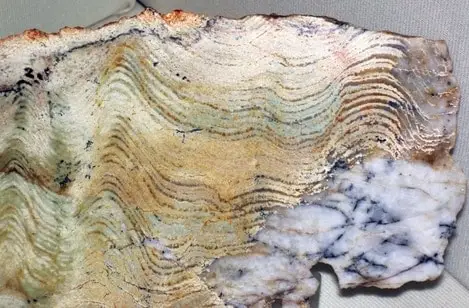
This event in history is referred to as the Great Oxidation Event (GOE). The rapid multiplication and diversification of cyanobacteria is also thought to have led to the decline of anaerobic bacteria which had dominated the ecosystem until then. The geological changes accompanying the rise in oxygen led to the evolution of eukaryotes through the last universal common ancestor (LUCA).
Yet, the contribution of cyanobacteria does not end there. Over 1.5 million years ago, an endosymbiotic relationship between cyanobacteria and unicellular eukaryotes led to the formation of chloroplasts. As a result, oxygenic photosynthesis spread among eukaryotes, resulting in diverse species of algae and plants. Subsequent endosymbiotic relationships between complex eukaryotes and cyanobacteria is thought to have led to the formation of more complex plastids. Cyanobacteria are thought to have evolved very slowly, as modern cyanobacteria continue to bear great similarity to their fossilized ancestors.
Classification of Cyanobacteria
The classification of cyanobacteria has varied with the elucidation of their morphology and characteristics. Until the end of the 20th century, cyanobacteria were recognized as algae and thus followed the International Code of Botanical Nomenclature.
However, with the discovery of the prokaryotic nature of cyanobacteria, they were reclassified under the Kingdom Monera, Division Eubacteria according to the International Code of Nomenclature for Bacteria. The oldest system proposed by Fritsch in 1935 merely grouped these bacteria into 11 categories based on morphology. Over time, diverse systems of classification were proposed by different algologists.
One of the more popular systems proposed by Fritsch in 1945 classifies over 2000 species of cyanobacteria into five orders (Table). Fritsch’s taxonomic order was later adapted by the National Centre for Biotechnology Information (NCBI) in 2004 and continues to be used till date.
ORDER | CHARACTERISTICS | EXAMPLE GENERA |
Chroococcales | Spherical cells that reproduce by binary fission or budding | Aphancocapsa |
Pleurocapsales | Spherical cells, colonies or pseudo-filaments that reproduce by forming endospores. | Chroococcidiopsis |
Oscillatoriales | Singular filaments. Do not form heterocysts or spores. | Lyngbya |
Nostocales | Filamentous cyanobacteria. Do form heterocysts. They only reproduce on one plane. | Anabaena |
Stigonematales | Filamentous | Mastigocladus |
Cyanobacteria can also be classified based on their ecological characteristics. These include:
- Mat-forming species which form biofilms of cyanobacteria over solid surfaces or plants.
- Bloom Forming Species – Form large colonies in water bodies.
- Picocyanobacteria – Small cells.
Cyanobacteria Structure
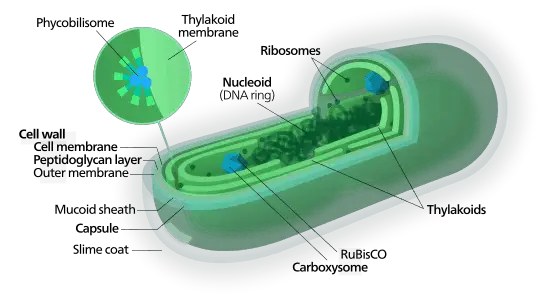
Cyanobacteria are morphologically diverse, ranging between spheroidal, rod-shaped, filamentous or tubular. Although cyanobacteria are unicellular, in nature they often cluster together to form colonies of filaments, sheets or spheres. Cyanobacteria vary in size between 0.5 (Species: Phlorococcus) to 100 micrometers (Species: Oscillatoria). However, they are typically much larger than other bacterial.
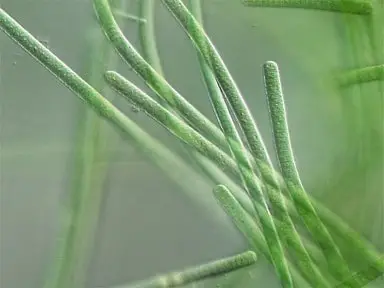
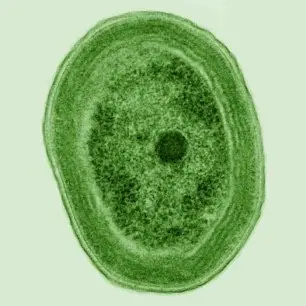
Modern cyanobacteria are enclosed with a peptidoglycan layer, resembling most other prokaryotes. The bacterial membrane can be further divided into an outer membrane and an inner membrane, enclosing a periplasmic space between them. The outer membrane is also typically perforated with pores which allows transport of molecules between the bacterium and its environment.
The bacterium or its colony may also be enclosed by a transparent or pigmented mucoidal envelope called the sheath. The external sheath is often composed of diverse monosaccharides including pentoses, hexoses, methyl sugars and amino sugars. The protective sheath has also aided in preserving the morphology of cyanobacteria in fossil records. In addition to a complex external membrane system, cyanobacteria contain internal structures which assist in photosynthesis.
The folded or coiled internal membranes called thylakoids (much like the structures in chloroplasts) are double membrane units which host the two photosynthetic systems and corresponding light absorbing pigments (carotenoids, phycobilin, chlorophyll) in its intermembrane space.
The folded network allows for a much greater surface area thus increasing the efficiency of light absorption and photosynthesis. The thylakoids may be arranged in diverse patterns (parallel, radial, columnar or disordered) based on both the species of cyanobacteria and the availability of light.
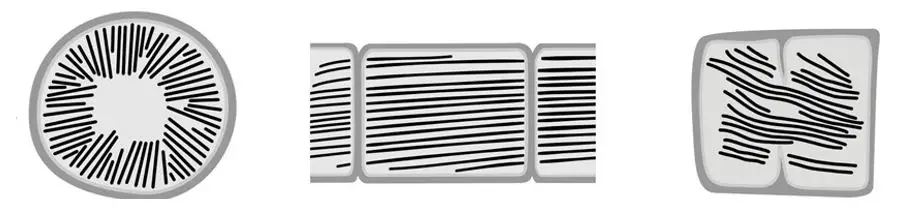
In addition to the thylakoid membrane, the stroma is also littered with enzymes, lipids and protein complexes which aid photosynthesis. These are often stored in specialized storage structures such as carboxysomes, cyanophycin vesicles and glycogen granules. Some bacteria may nano-compartments filled with gas called gas vesicles.
These structures increase buoyancy, thus helping the bacteria float in aqueous environments. However, like typical prokaryotes, these bacteria do not contain a well-defined nucleus. Instead, cyanobacterial DNA floats in the cytoplasm as a tightly coiled nucleoid.
Did You Know?
Scientists broadly categorize bacteria into two strains; gram positive and gram negative based on the components that make up their cell wall. The Danish Bacteriologist Hans Christian Gram developed a unique staining method using two dyes; crystal violet and safranin.
Gram positive bacteria which contain thicker peptidoglycan cell walls retain the crystal violet dye and thus, appear dark blue-purple under the microscope. In contrast, gram negative bacteria have thinner peptidoglycan layers which fail to retain the dye. Staining these cells with the counter stain safranin leaves them with a pink color under the microscope. Based on the cell morphology we just discussed, can you guess which category cyanobacteria belong to? That’s right most cyanobacteria are gram negative.
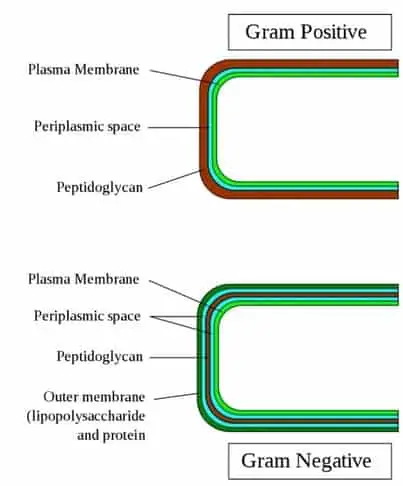
Cyanobacteria Reproduction
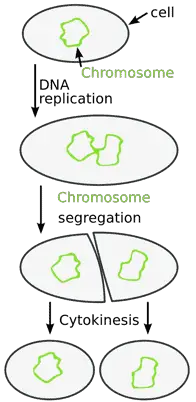
Cyanobacteria reproduce asexually by binary fission, multiple fission, budding or fragmentation. Unlike other bacteria, cyanobacteria display extraordinary diversity in their means of reproduction. Some common characteristics among all reproductive methods include the doubling of cell contents, formation of separating wall (septa), and finally, separation of two daughter cells.
In favorable conditions, cyanobacteria divide rapidly to form colonies. Some species under optimal conditions can double their population in times as short as several hours. Although their ability to rapidly divide is an ecological advantage, cyanobacteria have survived for millions of years due to their ability to survive and reproduce under harsh conditions.
When conditions are unfavorable for growth, active cyanobacterial cells differentiate into climate resistance spores. In harsh environments, cyanobacteria differentiate into thick-walled, dormant cells surrounded by a protective environment, called akinetes.
In contrast, when these bacteria are exposed to intolerant anaerobic conditions, they develop a thick walled envelope enclosing specialized enzymes for nitrogen fixation- called heterocysts. Some filamentous species also form multiple spores during unfavorable conditions. When these spores find favorable environments, they become enlarged and divide by binary fission.
Motility of Cyanobacteria
Cyanobacteria lack specialized structures for transport such as flagella, yet they display a remarkable capacity for motility. Most species of cyanobacteria move by gliding – the movement of an organism across solid or semisolid surfaces using propulsion mechanisms. The bacteria may also secrete slime which facilitates the gliding motion.
Furthermore, scientists have discovered sensory systems on cyanobacteria walls. These can sense and respond to light and chemical stimuli by initiating a gliding movement towards the stimulus. The sensory systems in cyanobacteria are so advanced when compared to its fellow prokaryotes that they can detect even small changes in the intensity and angle of light.
When larger colonies of cyanobacteria wish to move, they form motile filaments called hormogonia. These tapered filaments branch away from the bulk of the biomass towards new territories. Cells found in a hormogonium are typically thinner than vegetative cells. When a hormogonium finds an appropriate territory, it tears away from the parent colony, thus resulting in the formation of a new colony.
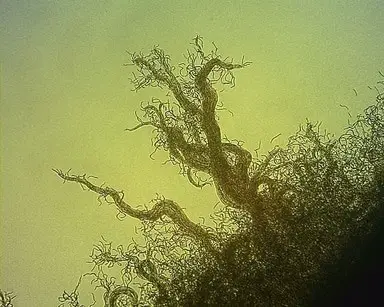
Cyanobacteria are a very adaptable species, capable of surviving in a wide range of water (freshwater, brackish water, oceans, hot springs), terrestrial (nutrient rich soil, desert, glaciers) and symbiotic relationships (with plants, lichens and animals). In fresh water, they may be found in the benthic zone or as part of the plankton. In land, they prefer moist surfaces such as rocks or tree bark where they grow as a cushy layer.
They also enter into some interesting relationships with animals in order to survive in harsh environments. For example, they can be found in the fur of sloths where they provide the anima with camouflage. Cyanobacteria have also been found in the harshest of environments where most other life cannot survive.
Cyanobacteria can grow in deep caves without sunlight and mountaintops exposed to high concentrations of solar radiation. Thermophilic cyanobacteria have been found to thrive in hot springs of temperature 73oC. Cyanobacterial blooms or colonies are a vital cornerstone of aquatic ecosystems.
By transforming light from the sun into organic nutrients, these bacteria are the primary producers in many water bodies. In terrestrial ecosystems, these bacteria strengthen the soil by preventing erosion and helping retain water. Furthermore, by fixing nitrogen from the atmosphere, they play a vital role in the geological development of both aqueous and terrestrial environments.
Did you Know?
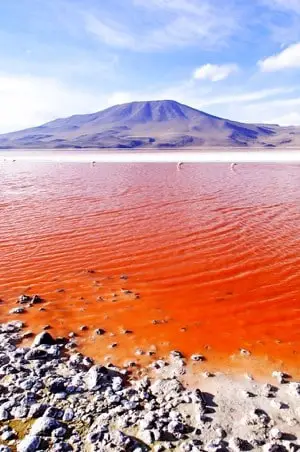
The ‘red’ sea (Greek: Erythra Thalassa) is a seawater inlet of the Indian ocean lying between Africa and Asia. Located southward of Egypt, the sea earned its name due to its transient changes in color. Although the water is blue-green during most parts of the year, it occasionally changes to a blood red. We now know that the change in color can be attributed to blooms of Trichodesmium erythraeum, a species of cyanobacteria. These organisms are rich in phycoerythrin pigments which render the sea with a reddish-brown color.
Cyanobacteria Metabolism
Cyanobacteria play a vital role in the ecosystem by sequestering carbon-dioxide, fixing nitrogen and releasing oxygen. The bacteria perform these function through two important metabolic pathways.
Photosynthesis: Cyanobacteria are the only prokaryotes capable of performing photosynthesis. Light energy or chemical energy is used to charge the electron transport chain, through the protein complexes present in the thylakoid membrane. The electron transfer catalyzes the reduction of NADP to NADPH, thus driving synthesis of the energy rich molecule- ATP.
Nitrogen Fixation: Cyanobacteria can fix nitrogen in the soil by converting gaseous nitrogen. In order to carry out this process, cyanobacteria are equipped with a specialized nitrogenase enzyme complex. Interestingly, nitrogen fixation is typically inhibited by oxygen. However, cyanobacteria are the only oxygenic microorganisms on Earth that are capable of nitrogen fixation.
The bacteria achieves this by both spatially and temporally separating photosynthesis and nitrogen fixation. While photosynthesis typically occurs in the day, nitrogen fixation occurs during the night. Spatial separation occurs when cyanobacteria differentiate into specialized heterocysts which carry out nitrogen fixation.
Commercial Uses of Cyanobacteria
In addition to the ecological importance of cyanobacteria, these microorganisms have also been exploited for commercial and industrial use. These include:
- Industrial production of amino acids
- Nutrient-rich cyanobacteria are used to produce a protein and vitamin-rich food supplement called ‘Spirulina’.
- Used in agriculture as fertilizers for their ability to fix nitrogen.
- Interestingly, cyanobacteria is currently being explored for use as a renewable energy source.
Cyanobacterial Toxicity
Although cyanobacterial metabolism is advantageous to the ecosystem, their uncontrolled division can prove toxic. Cyanobacterial blooms manifest as greenish scum, clogging water filtration systems, using up nutrients, and leaving the water with bad odor. Several species of cyanobacteria also secrete cytotoxins, neurotoxins, endotoxins and hepatotoxins. Collectively the toxic compounds secreted by cyanobacteria are referred to as cyanotoxins.
Although these bacteria are not classified as true pathogens (in that they do not invade the animal/human body), the ingestion of their toxins can cause several illnesses. Farm animals are often killed by drinking poisoned water from water bodies contaminated with cyanobacteria. Some human illnesses that can be caused by these bacteria include gastroenteritis, liver damage, pneumonia, sore throat, eye and skin infections. It has also been hypothesized that chronic exposure to cyanotoxins can lead to liver cancer. Consequently, the treatment and management of cyanobacteria in drinking water sources is of vital concern for countries around the world.
References
- Demoulin, C.F., Lara, Y.J., Cornet, L., François, C., Baurain, D., Wilmotte, A., Javaux, E.J., 2019. Cyanobacteria evolution: Insight from the fossil record. Free Radic Biol Med 140, 206–223. https://doi.org/10.1016/j.freeradbiomed.2019.05.007
- Garcia-Pichel, F., 2009. Cyanobacteria, in: Schaechter, M. (Ed.), Encyclopedia of Microbiology (Third Edition). Academic Press, Oxford, pp. 107–124. https://doi.org/10.1016/B978-012373944-5.00250-9
- Kumar, J., Singh, D., Tyagi, M.B., Kumar, A., 2019. Chapter 16 – Cyanobacteria: Applications in Biotechnology, in: Mishra, A.K., Tiwari, D.N., Rai, A.N. (Eds.), Cyanobacteria. Academic Press, pp. 327–346. https://doi.org/10.1016/B978-0-12-814667-5.00016-7
- Percival, S.L., Williams, D.W., 2014. Chapter Five – Cyanobacteria, in: Percival, S.L., Yates, M.V., Williams, D.W., Chalmers, R.M., Gray, N.F. (Eds.), Microbiology of Waterborne Diseases (Second Edition). Academic Press, London, pp. 79–88. https://doi.org/10.1016/B978-0-12-415846-7.00005-6
- Shestakov, S.V., Karbysheva, E.A., 2017. The origin and evolution of cyanobacteria. Biol Bull Rev 7, 259–272. https://doi.org/10.1134/S2079086417040090
- Singh, J.S., Kumar, A., Rai, A.N., Singh, D.P., 2016. Cyanobacteria: A Precious Bio-resource in Agriculture, Ecosystem, and Environmental Sustainability. Front. Microbiol. 7. https://doi.org/10.3389/fmicb.2016.00529
- Sivonen, K., 2009. Cyanobacterial Toxins, in: Schaechter, M. (Ed.), Encyclopedia of Microbiology (Third Edition). Academic Press, Oxford, pp. 290–307. https://doi.org/10.1016/B978-012373944-5.00005-5
- Vachard, D., 2019. Cyanobacteria, in: Reference Module in Earth Systems and Environmental Sciences. Elsevier. https://doi.org/10.1016/B978-0-12-409548-9.11843-3
- Vincent, W.F., 2009. Cyanobacteria, in: Likens, G.E. (Ed.), Encyclopedia of Inland Waters. Academic Press, Oxford, pp. 226–232. https://doi.org/10.1016/B978-012370626-3.00127-7