The nerve cell, also called the neuron, is a specialized cell capable of transmitting electrical signals. Sensory inputs originating from the environment are transmitted through the length of the neuron and the spaces between them (synapse), thus facilitating communication between diverse parts of the body and its surroundings.
The cells that make up living things are complex, versatile systems, capable of diverse functions. Among these varied cell-types, nerve cells – the cells that make up the brain are considered the most proficient.
The animal brain has been deemed the most complex machine on earth, capable of functions even a computer cannot replicate. The brain derives this ability from millions of nerve cells working together in unison to perform complex signaling functions.
Neurons form the fundamental building block of the nervous system, including the brain and the spinal cord. Most members of the animal kingdom, except sponges and Trichoplax, contain some form of neuron. In more complex organisms, the nervous system can be categorized into distinct central and peripheral nervous systems.
The number of neurons in an organism can vary widely between species. A typical human brain contains anywhere from 85 billion to 200 billion neurons. For comparison, the jellyfish (Hydra vulgaris) contains only about 5,600 neurons. The process by which neurons are generated from stem cells is called ‘Neurogenesis’.
Neural stem cells divide rapidly before fully differentiating to form neurons which can no longer divide. In most animals, neurons are only generated during brain development in the embryo and during childhood. As the animal reaches adulthood, neurogenesis largely comes to a halt.
More recent studies confirm the presence of neurogenesis in the adult brain, but only very specific neurons in the hippocampus and olfactory lobe are capable of dividing. However, this remains a largely contentious issue among scientists.
DID YOU KNOW?
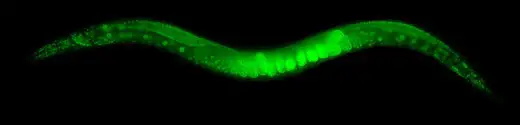
The roundworm (Caenorhabditis elegans) is the only organism whose entire nervous system consisting of 302 neurons and 7000 synapses has been mapped from start to finish.
The map called the ‘connectome’ which lays down the function of each nerve cell, has helped scientists interpret how the roundworm’s brain processes information. Neuroscientists are now calling for a similar connectome to be established for the human brain.
However, with 200 billion neurons, you can see why this would be a monumental effort!
Structure Of The Neuron
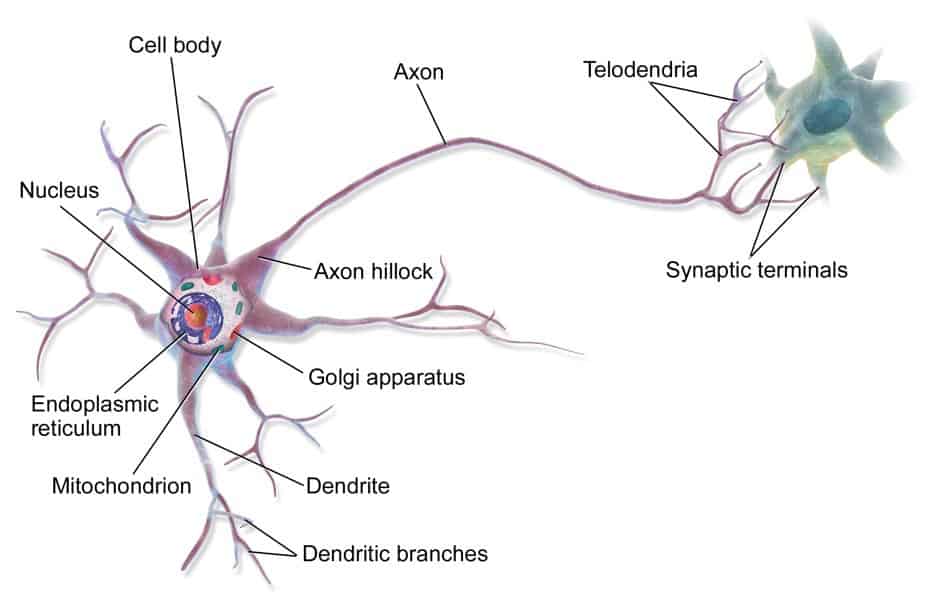
A typical nerve cell contains a cell body or soma from which two or more elongated fibers emerge. In this manner, a neuron largely resembles a tree and its branches. These branch-like extensions are called dendrites, which receive chemical signals from neighboring neurons.
The point at which one part of a nerve cell communicates with a neighboring cell is termed the synapse. Most higher-level organisms also contain a single elongated fiber called the axon which is highly specialized for rapid electrical transfer.
The size and shape of the neuron can vary depending on the organism and the function it is specialized for. While human motor neurons can extend up to one meter, giraffes have single axons that stretch several meters long. Likewise, the soma of the neuron can vary in diameter between 4 to a few hundred micrometers.
Some neurons have a limited number of dendrites that extend from the cell body. However, neurons that are specialized for rapid message transfer may have multiple networks of dendrites that connect diverse tissues.
The neurons of the Central Nervous system in humans, for example, can communicate with over 1000 neurons in a single second, therefore they are typically equipped with a complicated network of long dendrites.
Dendrites
These are cellular extensions which form the input point for neurons. Although dendrites are typically shorter than axons, they are highly complex in structure. Biologists believe that the unique tree-like shape evolved in order to provide a high surface-area within which signals can be transferred.
In some higher-level organisms, dendrites are specialized with distinct regions called dendritic spines which provide discrete reception sites for the incoming signal. The traditional view of nerve communication assumes dendrites are the singular input source in the neuron.
In contrast, even the soma may receive signals. Furthermore, some lower-level organisms do not have axons at all. In these organisms, the dendrites act as both an input and output source.
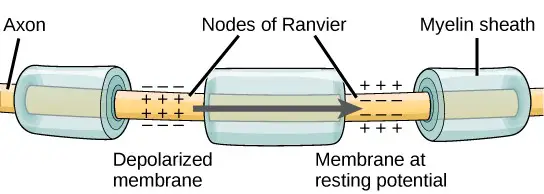
Axon
The axon is an elongated fiber that emerges from the cell body of the neuron. Although typically larger than the soma, the length of the axon can vary widely between species and neuron types. The primary function of the axon is to transmit electrical signals to and from the soma.
For this purpose, some axons are specialized with an insulating sheath of fat cells called the myelin sheath. Like typical plasma membranes, the myelin sheath is composed of 80% lipid and 20% protein. These concentric circles of protein and lipid ensure the signals are transmitted without loss. In some points, the myelin sheath is interrupted by non-insulated gaps called nodes of Ranvier.
As the electrical impulse jumps from node to node, it speeds up, thus ensuring rapid transfer. In some parts of the body, several axons may be bundled together to form a singular transmitted unit called tracts. The point at which the axon leaves the soma is called the axon hillock.
Neurons receive input signals all the time, however, not all inputs become functional signals. The axon hillock is equipped with a dense volume of voltage-dependent sodium channels which controls which signals will pass through the axon and which signals will be terminated at the soma.
The farthest end of the axon is called the axon terminal which forms a synapse with other neurons or cells. At the terminal, the electrical signal moving through the neuron is converted back into a chemical signal in the form of neurotransmitters.
Soma
The somais the central structure of the neuron which houses the nucleus and DNA. A nucleus can vary in diameter between 3-18 micrometers and is typically enclosed within a double-membrane. Unlike other structures in the neuron, the soma is not specialized for active transmission of signals.
Instead, the soma primarily serves to maintain the neuron. The soma is also the primary site of protein synthesis. In addition to the DNA, the soma is equipped with typical cell organelles including the Golgi apparatus and mitochondria. Some neurons also contain clumps of rough endoplasmic reticulum called Nissl bodies.
Cell Membrane
Like all cells, the neuron is also enclosed within a plasma membrane composed of lipid bilayers and embedded proteins. In most cells, the cell membrane serves to protect the organelles from environmental factors.
However, in neurons, the plasma membrane is equipped with electrically active membrane proteins that pump ions in and out of the neuron. The movement of electrically charged ions creates a voltage difference across the membrane; and in this way chemical signal received at the synapse is converted into an electrical signal which can move through the body of the neuron.
DID YOU KNOW?
Only some cells of the nervous system are neurons. Neuroglia are more simplified nerve cells present in most vertebrates and invertebrates. Initially, neuroglia were thought to possess connective cells that provide structural support to neurons.
However, neuroglia are adapted to serve multiple functions in the nervous system. Unlike neurons, neuroglia lack axons. Furthermore, unlike neurons, neuroglia can divide continuously throughout their lifetime. Most vertebrates contain three distinct types of neuroglia; astrocytes, oligodendrocytes and microglia.
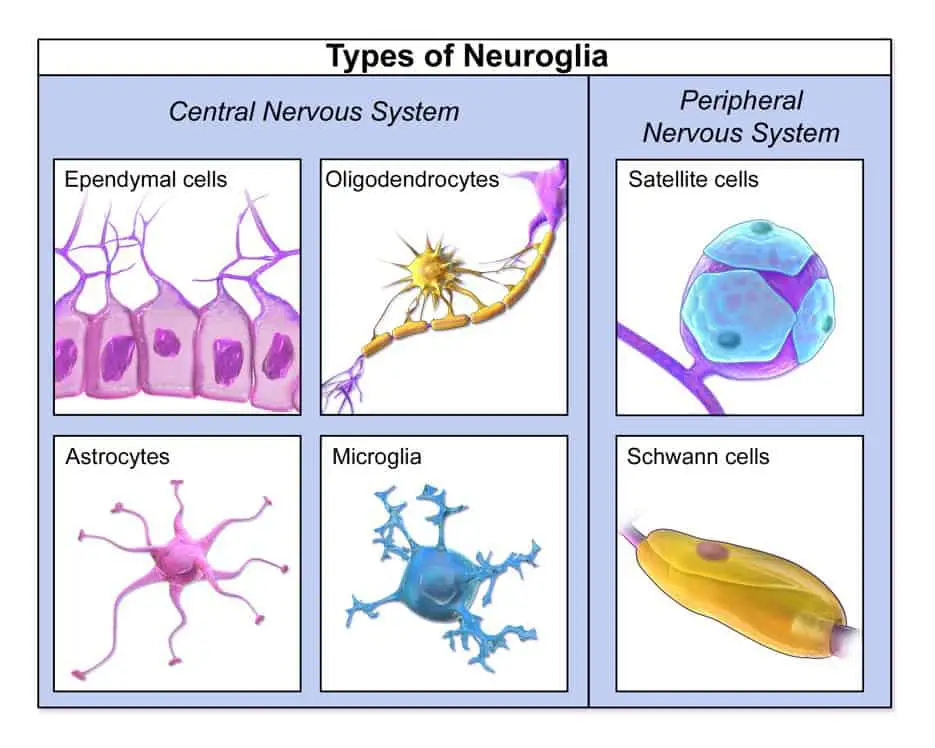
Function Of The Neuron
Neurons perform diverse functions in the body. Sensory neurons respond to stimulus such as light, sound and touch. In contrast, motor neurons regulate the movement of muscles in response to stimulus.
The primary function of the neuron is to transmit information both within and between neurons. The information being transmitted can take the form of both electrical signals and chemical messengers.
However, all neurons follow a singular principle of transmission which is termed the all-or-none principle. Unlike other signaling receptors which can transmit signals of varying strength depending on how they are stimulated, activated neurons produce a steady state of signals.
The dendrites receive information both from other neurons and cell types which is subsequently passed down through the cell body into the axon.
Transmission At The Synapse
Information reaches the synapse (or the point of connection between a dendrite and its surroundings) from neighboring neurons, other cells, or the environment. Several stimuli can trigger or activate a neuron, including environmental signals such as pressure, chemical transmitters such as neuropeptides, and electrical potential. Beyond this point, the information may be converted into electrical current or chemicals.
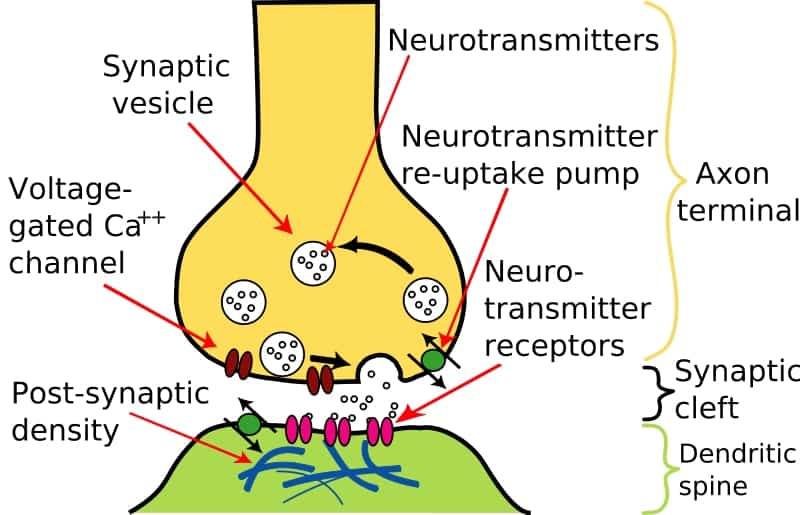
Electrical transmission of impulses take place through simple gap channels embedded in the membrane of the neuron. Adjacent neurons or neuroglia are linked together by gap channels. Diffusion of ions such as Calcium(Ca++), Potassium (K+), Sodium (Na+) or Chloride (Cl-) through these channels transmit electric potential rapidly without any delay.
Some gap junctions allow movement of ions with and against the gradient. However, some specialized gated channels are highly regulated to allow only the movement of specific molecules. Electrical transmission is far less common than chemical transmission and is only seen in invertebrates and lower vertebrates.
Chemical transmission of impulses begin when the action potential reaches the axon terminal. The change in voltage opens the voltage-gated ion channels, allowing an influx of Ca++ ions. This causes the vesicles at the terminal to release specialized chemical messengers called neurotransmitters through a process called exocytosis.
These chemical molecules are specialized to travel across the synaptic gap to activate receptors on an adjacent neuron or cell. After the signal is transferred, the neurotransmitters are absorbed back by the neuron by a process called reuptake. To date, more than 100 different types of neurotransmitters have been identified including acetylcholine, endorphins and dopamine.
Transmission In The Neuron
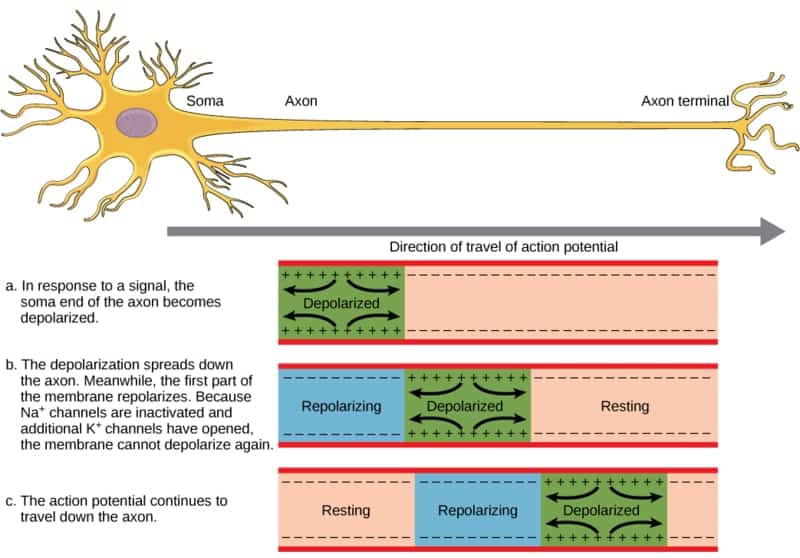
Transmission of the impulse through the neuron can be broadly categorized into three distinct event zones; before the action potential, during the action potential and after the action potential.
When a neuron is in resting or polarized state and not actively transmitting information, the neuron is negatively charged compared to the positively charged environment outside the cell. The difference in charge is maintained by a varying concentration of electrically charged ions such as Ca, Na, K or Cl. At the resting state, Na and K ions cannot pass through the membrane.
Similarly, Cl ions inside the neuron cannot escape. The neuron uses active transport to maintain this polarized state, meaning for every two potassium ions that enter the neuron, three sodium ions are expelled to maintain the charge. At this point, the resting potential of the neuron is defined as the difference in voltage between the inside and the outside. For a typical neuron, this is between -60 to -75 millivolts.
The action potential reaching the neuron may be stimulatory or inhibitory. An inhibitory signal tends to make the neuron more negative than the polarized state by a process called hyperpolarization. In contrast, a stimulatory signal makes the neuron less negative by a process called depolarization.
A stimulatory impulse causes the sodium channels to open causing an influx of sodium ions. When depolarization reaches a certain threshold, an action potential is generated, which subsequently travels down through the axon. The reverse polarity which measures the difference in voltage inside and outside a depolarized neuron is about +20 millivolts.
Immediately after the electrical impulse is transmitted, the sodium channels close. Following sodium inactivation, potassium channels open causing an expulsion of K+ ions outside the neuron. This process called repolarization causes the neuron to return to its previous polarized state.
Once a neuron is repolarized, there is a small amount of time called the absolute refractory period during which the neuron remains in resting state. During this time, the neuron can only be activated by a signal that is stronger than the previous signal.
Types Of Neurons
Neurons can be categorized differently based on diverse factors such as structure, function or anatomy. Here we describe the most common types and classifications of neurons.
Structural Classification Of Neurons
Neurons vary diversely in morphology, size and shape. However, based on the number of transmitting process extending from the cell body or soma, neurons are classified into four distinct categories.
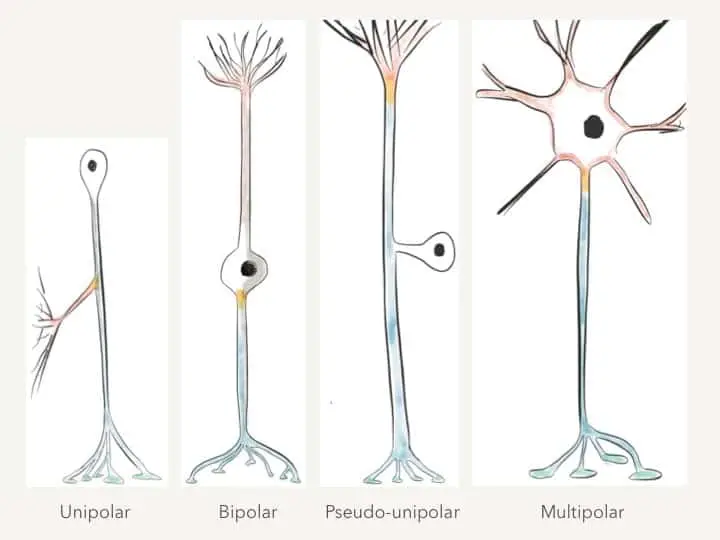
- Unipolar Neuron – These neurons only contain a single structure extending from the soma.
- Bipolar Neuron – These neurons contain both an axon and one dendrite extending from the soma.
- Multipolar Neuron – These neurons contain a single axon and multiple axons extending from the soma.
- Pseudounipolar Neuron – Although pseudounipolar neurons contain a single structure extending from the soma, they later branch into two.
Functional Classification Of Neurons
Neurons can serve diverse functions in the body. While sensory neurons typically respond to external stimulus such as light, sound and touch, motor neurons regulate the contraction and extension of muscles.
Based On Direction Of Information Transfer
- Afferent Neurons – Also called sensory neurons. They transmit information from tissues and organs to the central nervous system.
- Efferent Neurons – They transmit information from the central nervous system to other tissues and organs.
- Interneurons – They connect neurons with each other.
Based On The Type Of Neurotransmitters Released At The Axon Terminal
- Cholinergic Neurons – Release acetylcholine.
- Gabaergic Neurons – Release gamma aminobutyric acid.
- Glutamatergic Neurons – Release glutamate.
- Dopaminergic Neurons – Release dopamine.
- Serotonergic Neurons – Release serotonin.
- Histaminergic Neurons – Release histamine.
Evolution Of The Neuron
The evolution of complex neural networks from primitive unicellular organisms that first inhabited the earth has long been of interest to scientists. However, phylogenetic studies of nervous systems have been complicated by multiple factors.
Primarily, there remains the question – what differentiates a nerve cell from other cells? While nerve cells have fundamentally been defined by their ability to recognize and respond to stimulus, several non-nervous cells have also been shown to perform this function.
For example, several species of Jellyfish contain epithelial skin cells which can transmit electric signals in response to movements in their surroundings. Secondly, some neurons found in invertebrates cannot transmit electric potential at all!
Finally, epithelial, endothelial, secretory and muscle cells in higher level organisms resemble nerve cells with their ability to generate and transmit electric potential by secreting chemicals. These compounding factors led scientists to believe that neurons may have evolved more than once, so to speak.
Throughout evolution, organisms have gained and lost neurons in accordance with evolutionary advantage. The Trichoplax provides a prime example of this phenomenon. In these flat, multi-celled organisms, primitive neurons may have differentiated into epithelial cells capable of sensing and responding to stimulus.
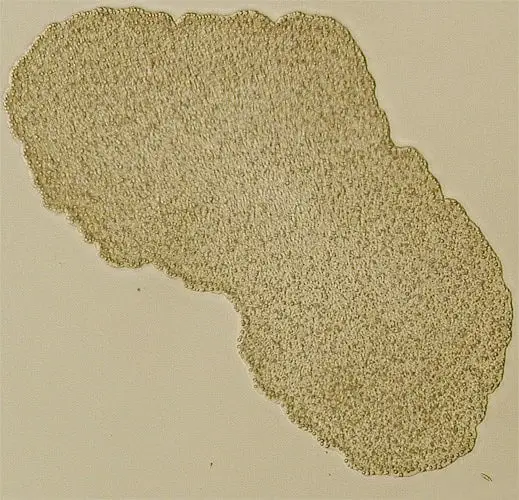
Fossils from the Precambrian period, dating about 550-560 million years ago provide the earliest evidence for the evolution of the nervous system. It is believed that during this time, animals began to prey on each other, travelling greater distances.
As a result, animals in this period developed systems that coordinated all functions and movement of the body to enable quick reflexes and defenses. Predatory pressure during this period is thought to have favored the evolution of cells capable of rapidly transmitting signals resembling the behavior of a neuron.
Voltage-gated channels in early prokaryotes and eukaryotes which allow for transport of specific molecules based on charge, are believed to have been the precursors for neurons. In fact, some modern bacteria continue to use these channels to communicate with the environment.
However, as larger and faster animals evolved, they would have needed more streamlined cells capable of communicating with one another. Although cell receptors are capable of recognizing and binding unique compounds called ligands to trigger intracellular signaling, this cascade is relatively slow.
This is thought to have led to the development of the cells specialized for rapid conduction. These early neurons were probably neuromuscular, allowing for multiple muscles in the body to move in coordination. However, as animals evolved more specialized organs, these neurons could have become more specialized for functions such as vision.
When Was The Neuron Discovered?
By the 17th century, experimental biology had definitively demonstrated that all living things were made up of cells. However, the cells that make up nervous tissue remained undiscovered. At the time, it was conjectured that the nervous system may be exempt to the cell theory.
However, in the late nineteenth century, examination of nervous tissue under high power microscopes helped identify cell bodies with diverging projections. This led the German histologist Joseph Gerlach’s theory of the reticulum – according to which the nervous system consisted of a web of continuous fibers.
In 1873, the Italian physician Camillo Golgi developed a unique staining method which allowed visualization of dead tissue under high detail. He hardened a piece of brain tissue in potassium dichromate before staining it with silver nitrate. This helped identify nerve cells as black figures against a yellow background.
They observed a long slender branch of tissue emerging from a smaller cluster of branching fibers. However, this structure did not fully contradict Gerlach’s theory of the reticulum.
In 1887, the Spanish neuroanatomist Santiago Ramon y Cajal used an advanced modification of Golgi’s staining method to further examine the nervous tissue. He identified that although nerve cells were often connected to each other through fiber like branching, they were distinct physical structures.
As a result, the ‘Reticulum’ theory was disproved and like all other living tissue, the nervous tissue was also shown to be made up of smaller cells. Using these observations, Cajal produced several detailed sketches of nervous tissue from a wide variety of animals and people.
To this day, Cajal’s drawings have proven so accurate that they are used to teach medical students. The term ‘neuron’ was finally coined in the year 1896 to refer to cells of the nervous tissue.
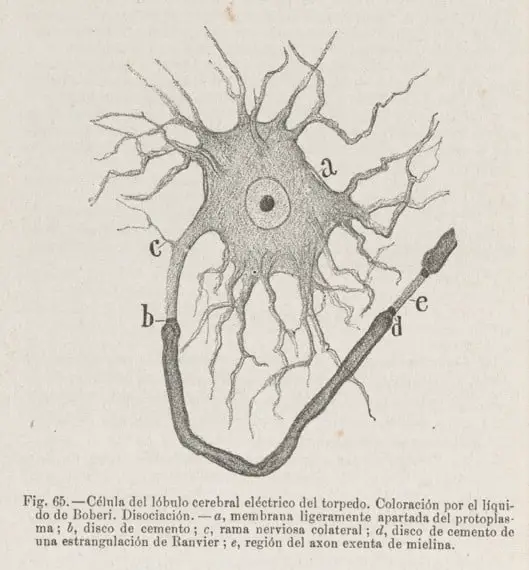
References
- Arendt, D., Bertucci, P.Y., Achim, K., Musser, J.M., 2019. Evolution of neuronal types and families. Curr Opin Neurobiol 56, 144–152. https://doi.org/10.1016/j.conb.2019.01.022
- Arendt, D., Tosches, M.A., Marlow, H., 2016. From nerve net to nerve ring, nerve cord and brain–evolution of the nervous system. Nat. Rev. Neurosci. 17, 61–72. https://doi.org/10.1038/nrn.2015.15
- Bentivoglio, M. Life and Discoveries of Santiago Ramon y Cajal. Nobelprize.org. 1998. http://www.nobelprize.org/nobel_prizes/medicine/laureates/1906/cajal-article.html
- Boorboor, S., Jadhav, S., Ananth, M., Talmage, D., Role, L., Kaufman, A., 2018. Visualization of Neuronal Structures in Wide-Field Microscopy Brain Images. IEEE Transactions on Visualization and Computer Graphics PP, 1–1. https://doi.org/10.1109/TVCG.2018.2864852
- Costandi, M. The discovery of the neuron. Neurophilosopy. 2006. http://neurophilosophy.wordpress.com/2006/08/29/the-discovery-of-the-neuron/
- Jabr, F., 2012. The Connectome Debate: Is Mapping the Mind of a Worm Worth It? [WWW Document]. Scientific American. URL https://www.scientificamerican.com/article/c-elegans-connectome/
- Kandel ER, Schwartz JH, Jessell TM 2000. Principles of Neural Science, 4th ed. McGraw-Hill, New York
- Kristan, W.B., 2016. Early evolution of neurons. Current Biology 26, R949–R954. https://doi.org/10.1016/j.cub.2016.05.030
- Mazzarello, P. A unifying concept: the history of cell theory. Nature Cell Biology 1, E13 – E15 (1999) doi:10.1038/8964
- Salio, C., Lossi, L., Merighi, A., 2011. Combined light and electron microscopic visualization of neuropeptides and their receptors in central neurons. Methods Mol. Biol. 789, 57–71. https://doi.org/10.1007/978-1-61779-310-3_3
- Schoonover, Carl. 2010. Portraits of Mind. Abrams.