The identification of bacteria is a very dynamic area of research. Bacteria are studied not only for the negative effects on our lives such as infections or food spoilage but also for the positive roles they play in our bodies and ecosystems. They are used in the food industry, genetic engineering, and biotechnology.
There are many ways to identify bacteria and some are more reliable than others but here are the 11 primary methods of bacterial identification:
- Gram Stain
- Endospore Stain
- Acid-Fast Stain
- Catalase and Oxidase Testing
- Probabilistic Identification
- Phenotypic Identification Systems
- Mass Spectrometry and Flow Cytometry
- Antibody Reactions
- Microarray
- Ribotyping
- Genotypic Molecular Identification Techniques
The advancement of the existing antibiotics and the discovery of the new ones are largely dependent on the advancement of the bacterial identification techniques. Equally, the speed of identification could be very critical, for instance in case of a novel disease such as the COVID-19. Though the COVID-19 is a viral infection, the principle of molecular (genetic) identification technique is the same.
These advanced molecular identification techniques are not limited by the type of microorganism. In fact, in the post Archaea: Ancient Microorganisms the same technique was used to discover a completely new domain of life called “Archaea”. In this post we will explore the different methods by which scientists used to identify bacteria.
Background
Humans being curious about their environment always prioritized the classification and identification of organisms. However, unlike animals and plants that possess good morphological characters, identifying the bacteria based on morphology is rather limited. Even then, bacteria were primarily grouped according to their morphological features and growth conditions such as cell shape (cocci: round, bacilli: rod-shaped, spirillum: spiral-shaped, etc.), presence or absence of flagella, arrangement of flagella, substrate (food) utilization, oxygen dependence (aerobic or anaerobic) and the growth pattern on solid media.
Apart from these, features such as sporulation, cell wall composition, antigenicity, biochemical activity, sensitivity to antimicrobial agents, and reactions to distinct biochemical markers are also used for the identification of bacteria.
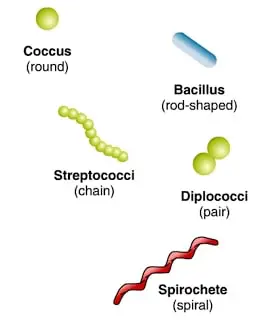
Intriguingly, different bacterial species produce distinct colony structures. The growth features on solid agar media include their shape (circular, irregular, rhizoid, etc.), size (the diameter of the colony), elevation (flat, convex, concave, etc.), surface (smooth, wavy, rough, etc.), margin (entire, curled, etc.), color (yellow, green, etc.), opacity (opaque, translucent or transparent), degree of growth (scanty, moderate or profuse) and nature (confluent, spreading or rhizoid). The traditional methods use these properties by either observation of the morphology of a single cell or a colony characteristic.
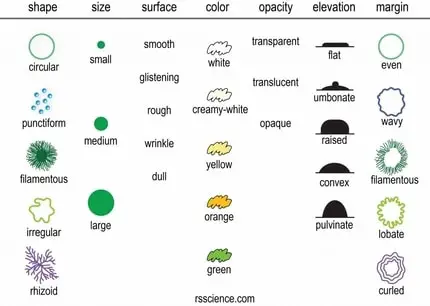
In the 16th century, Dutch microbiologist Antonie van Leeuwenhoek was first to see bacteria under a microscope. Light microscopy has been traditionally used for identifying bacteria but is often limited by inadequate resolution. Today, advanced scanning electron microscopy (SEM) coupled with high-resolution back-scattered electron imaging are used in the identification process.
1. Gram Stain
Though basic identification can be carried out using a light microscope, without staining, bacteria look colorless, transparent, and are very difficult to see. With improved coloring and contrast, the dyes overcome these problems by facilitating the cellular structure identification. In the 18th century, Hans Christian Joachim Gram used a combination of two dyes, crystal violet and safranin, to classify bacteria based on the cell wall structural properties.
The cell wall of Gram-positive has a higher peptidoglycan and lower lipid content than the Gram-negative. During the staining procedure, the Gram-positive bacteria retain crystal violet and appear blue. In contrast, Gram-negative lose crystal violet during the washing steps and hold only safranin to appear red. Bacterial species Bacillus and Escherichia are the example of the Gram-positive and Gram-negative respectively. After Gram staining, the morphological difference between a coccid and rod-shaped becomes much clearer under a compound light microscope with only 100X magnification.
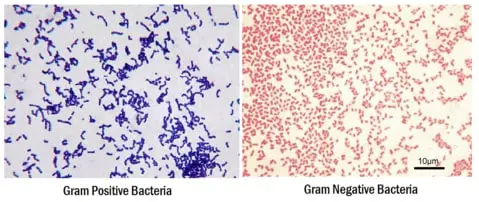
2. Endospore Stain
Analyzing the bacterial morphologies and the Gram stain is considered the first stage of identification. Spore production in Gram-positive rods such as Clostridium is an important survival feature. Therefore, identifying the spores using staining with malachite green and safranin dyes is another useful tool. This is also known as Schaeffer–Fulton stain or an endospore stain. In this, bacterial rods stain red while the spores stain green. The presence of spores is a clear indication for spore-producing Gram-positive bacteria.
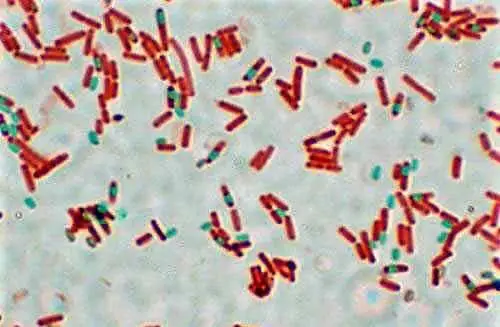
3. Acid-Fast Stain
Some bacteria such as mycobacterium,which can cause tuberculosis, render Gram-staining ineffective because their cell wall is extremely difficult to penetrate. The cell walls of these types of bacteria are made up of mycolic acid, fatty acid, and complex lipids which also makes them resistant to disinfectants.
In the year 1883, Dr. Franz Ziehl and the pathologist Friedrich Neelsen together developed an alternate staining for these bacteria which is now known as the Ziehl–Neelsen staining or Acid-fast staining. Acid-fast staining primarily utilizes a lipid soluble stain called carbolfuchsin which allows the stain to penetrate the cell wall of these types of bacteria. A decolorizer along with heat are added which removes the stain from all non-acid-fast cells, leaving the acid-fast cells stained.
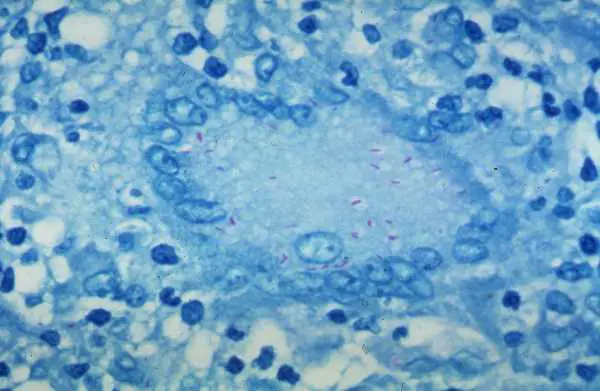
4. Catalase and Oxidase Testing
Traditionally, different biochemical methods have also been used to identify bacteria based on the enzymatic activity. These methods include the catalase or oxidase tests. A bacterial species with catalase activity produces bubbles of oxygen when hydrogen peroxide is added to the bacterial smear on a microscope slide. Bacterial species such as Staphylococci, Salmonella, and E. coli are catalase-positive, while Streptococcus is a catalase-negative. The oxidase test looks for the presence of enzyme cytochrome c oxidase in the bacteria.
5. Probabilistic Identification
Apart from these, bacteria utilize different carbon or nitrogen sources for energy, and this can also be used for probabilistic identification. Therefore, using the distinct culture media, specific bacterial species are identified. Mostly these tests also include a color indicator that changes color based on the change in pH (ex. methyl red test) or in the presence of a specific enzymatic activity. For instance, the Indole test looks for the enzyme tryptophanase that degrades tryptophan into indole which then reacts with Kovac’s reagent to produce a cherry red color.
6. Phenotypic Identification Systems
These days, a semi-automated phenotypic identification system such as Biolog is quite popular. It has been used to identify various lactic acid bacteria strains and is especially useful if a bacterial sample is within a fixed degree of similarity. It works on the process of elimination. For instance, if test 1 is positive and 2 is not, then one group of possible microorganisms is included, and another is excluded. Then the tests 3 and 4 are performed, and so on.
The resultant metabolic profile is compared with the appropriate Biolog database, containing the biochemical fingerprints of hundreds of Gram-positive and Gram-negative species. In 1989, the Biolog system was created to identify Gram-negative bacteria, but now its capability has been broadened to include the Gram-positive also.
7. Mass Spectrometry and Flow Cytometry
Another method is the analysis of cellular fatty acids by using gas chromatography. Here, identification is made based on the patterns of fatty acid esters. However, its popularity is going down and recently developed phenotypic methods such as mass spectrometry and flow cytometry have gained interest. Matrix-Assisted Laser Desorption Ionization Time-Of-Flight (MALDI-TOF) is a cutting-edge mass spectrometry instrument that identifies microorganisms based on characteristic biomarker protein fingerprints.
In this method, a single isolated colony or cell extract is bombarded with electrons for the ionizations of proteins. These are then separated based on their mass/charge ratio. Finally, the resulting spectrum, a protein fingerprint is matched against a database of known spectra. There is also an alternate mass spectrometry method, named PCR electrospray ionization MS (PCR-ESI/MS) which uses ionization of genetic material instead of proteins. The Flow cytometry uses serological (antibodies based) methods to analyze cells suspended in a liquid medium by light, electrical conductivity or fluorescence while individual cells pass through a very small opening.
8. Antibody Reactions
Clinically, previously identified bacteria can be easily identified by using “specific” antibody reactions in a serological test performed on a glass slide. In advanced Fluorescence In Situ Hybridization (FISH) method, binding of short fluorescence-labeled DNA or nucleic acid-mimicking PNA (peptide nucleic acid) probes to the ribosomes of infectious agents allows the identification of organism at genus or species level. Apart from these, the Antibiotic sensitivity test is another clinical method that identifies an organism based on its ability to grow on artificial nutrient media containing distinct antibiotics.
9. Microarray
Microarray is another method for microbial identification by the hybridization of pre-amplified microbial DNA sequences to arrayed species-specific oligonucleotide probes. Each probe contains a distinct dye that fluoresces upon hybridization and facilitates the detection and discrimination of different microbial samples on a single slide.
10. Ribotyping
The identification of bacterial species based on Ribotyping exploits sequence differences in rRNA. Organism DNA is digested with restriction enzymes to generate a unique combination of discrete-sized fragments (ribotyping fingerprint) for a particular bacterial species. This pattern is searched against databases that contain numerous patterns of different bacterial species.
11. Genotypic Molecular Identification Techniques
Novel DNA sequencing technologies (genotypic molecular identification techniques) are high-throughput and can generate huge amounts of genetic information with speed, accuracy, and efficiency. For instance, 16S rRNA gene sequencing provides a more detailed assessment of genetic traits compared to any other routine identification methods.
Takeaways
In a nutshell, the choice of a bacterial identification technique is based on several factors such as cost, speed, specificity, and need of a culture. How far one should go is decided by the nature of the inquiry. The traditional techniques are time-consuming, laborious, and suffer from culture variability due to challenging environmental conditions. Most important, a pure culture is a must which is nothing but the isolation of a single strain of bacteria from the mixed environment of organisms.
This is sometimes impossible in cases of an unknown species for which culture condition are also unknown. To evade these hurdles, advanced automated methods have been adopted which are not only quick but also more reliable. Recently, despite the cost factor, the 16S rRNA gene sequencing and mass spectrometry have emerged as preferred genetic techniques since these are neither limited by the laboratory growth nor the type of organism.
References
- “Bacterial Identification | AACC.Org.” Accessed June 21, 2020. https://www.aacc.org/publications/cln/articles/2012/may/bacterial-id.
- “Crash Course in Microbial Identification – Bitesize Bio.” Accessed June 21, 2020. https://bitesizebio.com/36644/methods-microbial-identification/.
- “Fluorescence in Situ Hybridization (FISH) in the Microbiological Diagnostic Routine Laboratory: A Review – PubMed.” Accessed June 21, 2020. https://pubmed.ncbi.nlm.nih.gov/28129707/.
- Biology Discussion. “Identification of Bacteria: 7 Steps,” May 16, 2016. https://www.biologydiscussion.com/bacteria/identification-of-bacteria-7-steps/30652.
- “Isolation and Identification of Two Bacterial Unknowns (Theory) : Microbiology Virtual Lab II : Biotechnology and Biomedical Engineering : Amrita Vishwa Vidyapeetham Virtual Lab.” Accessed June 21, 2020. http://vlab.amrita.edu/?sub=3&brch=76&sim=1109&cnt=1.
- Biology Discussion. “Isolation of Bacteria in Pure Culture,” May 16, 2016. https://www.biologydiscussion.com/bacteria/isolation-of-bacteria-in-pure-culture/30646.
- Phumudzo, Tshikhudo, Nnzeru Ronald, Ntushelo Khayalethu, and Mudau Fhatuwani. “Bacterial Species Identification Getting Easier.” African Journal of Biotechnology 12, no. 41 (October 31, 2013): 5975–82. https://doi.org/10.5897/AJB2013.12057.
- Sandle, Tim. “9 – Microbial Identification.” In Pharmaceutical Microbiology, edited by Tim Sandle, 103–13. Oxford: Woodhead Publishing, 2016. https://doi.org/10.1016/B978-0-08-100022-9.00009-8.
- Váradi, Linda, Jia Lin Luo, David E. Hibbs, John D. Perry, Rosaleen J. Anderson, Sylvain Orenga, and Paul W. Groundwater. “Methods for the Detection and Identification of Pathogenic Bacteria: Past, Present, and Future.” Chemical Society Reviews 46, no. 16 (2017): 4818–32. https://doi.org/10.1039/C6CS00693K.
- Zhu, Na, Dingyu Zhang, Wenling Wang, Xingwang Li, Bo Yang, Jingdong Song, Xiang Zhao, et al. “A Novel Coronavirus from Patients with Pneumonia in China, 2019.” The New England Journal of Medicine 382, no. 8 (February 20, 2020): 727–33. https://doi.org/10.1056/NEJMoa2001017.