Archaea are ancient, single-celled organisms which lack a nucleus and organelles. Morphologically similar to that of prokaryotes, archaea reproduce asexually by fission or budding and do not produce spores. Genetically archaea are closer to eukaryotes and have similarities in the fundamental cellular processes such as transcription and translation.
This evolutionarily unique position of archaea between the prokaryotes and eukaryotes makes them an intriguing group of microorganisms.
Archaea History
Until the 20th century, the primitive classification divided all the living things either as a plant or an animal. Later, with the evolution of microscopy, other miniature and microscopic organisms such as bacteria, fungi, and protists were found. To incorporate these organisms, there was a shift away from the prior, more primitive classification system, to an advanced kingdom system.
The prokaryotic kingdom represented bacteria and the other four eukaryotic kingdoms denoted plants, animals, fungi, and protists. This clear distinction was based on morphology and metabolic functions. The eukaryotes have a nucleus, cytoskeletons, and internal membranes while the strictly unicellular prokaryotic organisms lack these features.
In the late 1970s, with the understanding of gene coding DNA material, scientists discovered an entirely new group of organisms. The research group of Professor Carl Woese at the University of Illinois was exploring relationships among bacteria based on the similarity between their DNA sequences. Surprisingly, they found two distinctly different groups of organisms.
One group of organisms that lived at high temperatures, clearly identified as prokaryotes (bacteria) but the other methane-producing group of organisms failed to be classified with either prokaryotes or eukaryotes. This new information enthralled the scientific community. Due to the significant difference at the genetic level, Professor Carl Woese named this new group of organisms “archaebacterial”.
However, as he advanced his understanding, he felt that archaebacteria was still a misnomer. Hence, he finally shortened the name from archaebacteria to “archaea”. According to ancient Greek, the word archaea means “ancient things”.
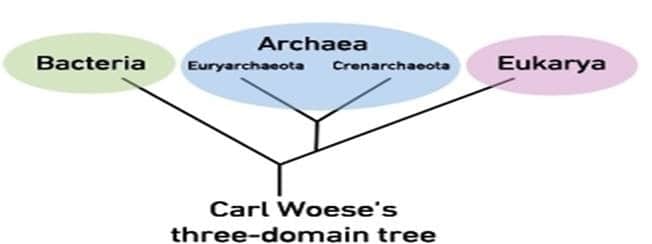
Fossil studies seem to indicate that prokaryotes are the most primitive and ancient life forms on earth. The very early prokaryotic cell fossils are almost 3.5 billion years old. It is important to note that prokaryotes do not have distinctive morphological features. Based on the morphologic features, most archaea are also quite similar to bacteria. This raises the questions on the true identity of the prokaryotic fossils.
The detection of the distinctive lipids from the fossils is much better evidence for identifying the class of organisms. Indeed, 2.7 billion years old lipid fossils have been found that are now believed to be of either archaean or eukaryotic origin. This certainly hints at the early presence of archaea along with prokaryotes.
Archaea Habitat
Archaea were originally isolated from extreme temperature environments. This led scientists to believe that they are predominantly in anaerobic environments and hot springs. Though, later archaea were also found in very cold, highly saline, acidic, and alkaline habitats. Gradually mesophile archaea were also discovered.
These grow in mild environments such as sewage, swamps, and soils. In oceans they are abundant members of the marine plankton and sea sediments. Symbiotic relationship between archaea and some marine animals is also evident, given that archaea have been isolated in the gut of some sea animals. Contrary to earlier beliefs, archaea are now thought to form a major part of global ecosystems.
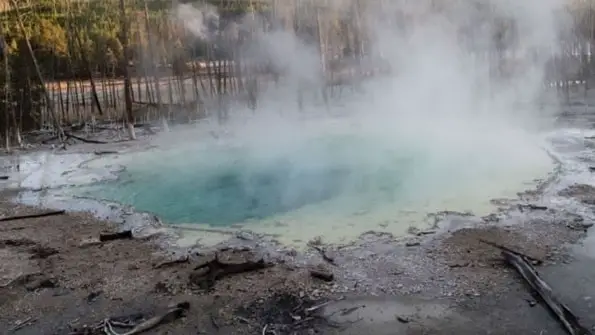
However, compared to bacteria, the overall physiological diversity within the archaeal organismsis limited. Archaea happily survive in extreme environments that are hostile to many other life forms. Extremophile archaea include halophiles, thermophiles, acidophiles, and alkaliphiles. The halophiles live in extremely saline environments and can actually outnumber bacteria when salinities are above 20%. The thermophiles grow best in places where temperatures are usually above 45 °C, like hot springs. The hyperthermophile archaea grow at temperatures above 80 °C.
In fact, it is an archaeon that holds the record for living at the highest temperature. The Strain 121 was named for its ability not just to survive, but to reproduce at 121 °C. Notably, for sterilization purposes, 121 °C is the temperature that is used to kill all forms of life. Before the discovery of Strain 121, no cells were known to survive at this temperature.
Now scientists believe that there could be archaeal species that can tolerate temperatures of up to 150°C. Similarly, acidophile archaea grow at pH 0, which is equivalent to swimming in the pool of concentrated sulfuric acid. One of the theories about early of life on earth suggests that microbes were transferred between planets through meteorites which, given the ability of these organisms to withstand extreme environments, seems add plausibility to the theory.
Some of the extreme habitats of archaea are similar to environments on Mars. However more research is necessary to understand these organisms and several archaeal organisms are difficult to culture making it challenging to learn more about them.
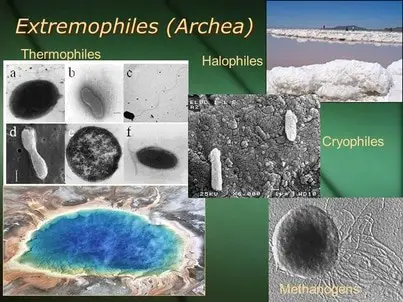
Archaea Energy Production
Archaea use a vast variety of metabolic chemical reactions. Based on these reactions, archaea can be grouped into nutritional groups. Lithotrophs obtain energy from inorganic compounds such as ammonia and sulfur. The other group, named phototrophs, uses light for energy but does not engage in typical oxygen-producing photosynthesis.
In these organisms, the protein bacteriorhodopsin that is functionally and structurally similar to the eye pigment rhodopsin is used to generate ATP (Adenosine triphosphate). However, since this reaction does not involve oxidation-reduction, no oxygen is generated. The archaea that thrive in anaerobic settings use a primitive form of metabolism similar to many of the other first free-living organisms.
Some archaea, known as methanogens, create methane from carbon dioxide and hydrogen, while others use organic carbon. These archaea are pretty common in the ocean and many freshwater habitats.
Taxonomic Classification of Archaea
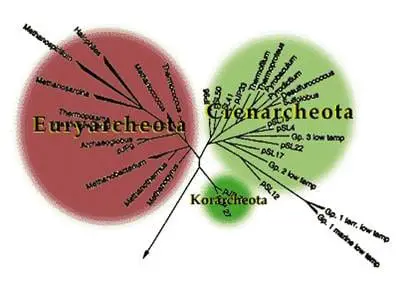
The classification of recently discovered life domain archaea is a rapidly moving area. The usual approach is to organize members into groups based on the similarity of their structural features. However, archaeal classification is heavily dependent on the sequence similarities between the highly conserved ribosomal RNA (rRNA) genes.
The logic is also relevant to the fact that their discovery was possible due to the presence of distinct ribosomal RNA genes that failed to match with either the prokaryotes or eukaryotes. The evolutionary relationship between the organisms is depicted as a phylogenetic tree. Notably, the phylogenetic trees are hypotheses and might not be definitive.
The pattern of branching in a phylogenetic tree reveals the path of evolution among the species from a series of common ancestor organisms. Theoretically, the most recent organism(s) from which all organisms of a clade evolved is known as the last common ancestor. Importantly, it does not signify the very first life of that clade on earth, but rather represent the organism for which living descendants are present.
Since in the beginning, Earth’s temperature was high, many believed that early life originated in a high-temperature environment. This was also supported by the fact that the last common ancestor of the bacteria and archaea is a thermophile and organisms that could tolerate cold environment appeared later in the evolutionary timeline. However, this hypothesis is now being challenged by the recent discovery of molecular sequences from archaea that lived in low-temperature environments. These also seem to be originating very early in an evolutionary (phylogenetic) tree.
According to Dr. Carl Woese, bacteria, archaea, and eukaryotes that represent the separate evolutionary lines possibly got separated at a very early time point during evolution. In genetics, vertical gene transfer is known as the acquisition of genetic material from an ancestor whereas lateral or horizontal gene transfer is the acquisition of genetic material from another species without being its offspring. Vertical gene transfer is possible using viruses or plasmids. Viruses, during infection, are capable of obtaining genetic material from one species and then transferring it to a completely unrelated host organism upon subsequent infection.
On the other hand, a plasmid is a small, extrachromosomal DNA molecule that is physically separated from chromosomal DNA and can replicate independently. These are found within the cells of organisms in all domains of life. In the beginning, when life evolved without a cell wall, the transfer of plasmids was possibly much easier. We now know that the relationship between archaea and viruses is nearly 2 billion years old. However, the mechanism of gene transfer in archaea is still poorly understood.
Nonetheless, this is now evident enough that the co-evolution of all three domains of life started at a very earlier stage. However, the extreme conditions that triggered the event are difficult to determine. The archaea domain is broadly ordered into three principal phyla, named euryarchaeota, crenarchaeota, and korarchaeota. However, only the members of euryarchaeota and crenarchaeota have been studied extensively.
The reason for this is that the members of korarchaeota do not have any cultured representatives to date. These were identified based on genetic molecular sequences. The advanced molecular biology tools now available allow the genome sequencing even from a single cell or a very minute sample of genetic material that can be obtained from a source site. The specific need for growing a culture for this purpose has become obsolete. Therefore, the korarchaeota is also considered a new branch of life that has significant implications for the early evolution and physiological diversity.
Further research of korarchaeota is still limited by the pure isolation of strains and unknown culturing conditions in the laboratory. The phylum euryarchaeota is highly diverse and includes methanogens, halobacteria, and some thermophile aerobes and anaerobes. These are separated from the other archaea members based on rRNA sequences and their unique DNA polymerase, an enzyme that helps in making copies of genetic material.
Initially, the crenarchaeota was thought to be sulfur-dependent extremophiles but recently the organisms that are abundant in the marine environment were also found based on their similarity of rRNA genes. Firstly, they were separated from the other members based on rRNA sequences and other physiological features, such as lack of histone proteins, although now some crenarchaea has also been found that have histones. These organisms stained Gram-negative and morphologically their cells are rod, cocci, or filamentous shaped. Until recently most cultured crenarchaea were of thermophiles or hyperthermophiles but now other members have also been identified.
It is important to note that archaea were discovered nearly 42 years ago. The classification is still in the initial stage as the knowledge is constantly growing and changing. The basic difference among the phyla, based on the habitat, metabolism, or morphology is continuously getting blurred as new fascinating members are being discovered. This is also changing the classification of archaea regularly.
Unique Features of Archaea
Archaea are without a cell nucleus or organelles. Morphologically, they are quite similar to bacteria. Some archaeal species come in amazing square-shaped cells. Similar to bacteria, archaeal cells can also aggregate to generate filamentous mass known as biofilms. Part of the function of this biofilm is to hold a large population of the organism together.
Despite of high visual similarity to bacteria, archaea possess genes and metabolic pathways that are more closely related to eukaryotes. Although archaeal flagella function like bacterial flagella, the flagella seems to have originated from a different ancestor. The bacterial flagellum is assembled by subunits moving up the central pore to the tip of the flagella while archaeal flagella are constructed from the addition of subunits at the base.
Similarly, bacterial cell walls contain peptidoglycan while archaea do not. This is a distinguishing feature between the archaea and bacteria. Similarly, there is a difference in lipid chemistry of the cell membrane of archaea and bacteria. In bacteria and eukaryotes, cell membrane fatty acids are linked by ester bonds to a molecule of glycerol. In archaea, the same bonding is carried out by an ether bond.
Although a few bacteria contain ether-linked lipids, there have been no archaea discovered that contain ester-linked lipids. Ether bonds being more stable than ester bonds probably assist archaea surviving in extreme environments. This also implies that archaea use different types of enzymes for the synthesis of the cell membrane.
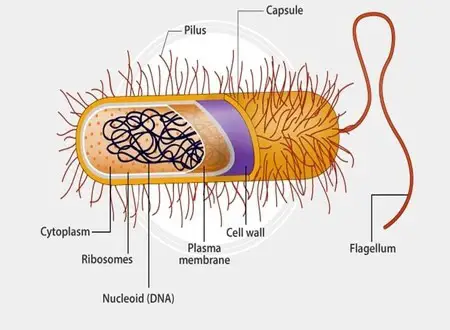
In archaea, the important enzymes such as DNA polymerase and RNA polymerases that respectively copy DNA or generate an RNA read seems to be the simpler versions of the eukaryotic counterparts. Similar to eukaryotes but unlike bacteria, the single circular chromosomes in archaea can have more than one origin of replication. In bacteria, RNA polymerase is made up of four chains of amino acids (polypeptides).
However, the RNA polymerase of archaea contains more than eight polypeptides whereas the RNA polymerases of eukaryotes have even higher (up to 12) number of polypeptides. The relative lengths of the polypeptides in archaeal RNA polymerase are also close to eukaryotes.
In archaea, the various features of the process of protein synthesis, including the machinery ribosomes, are also similar to eukaryotes. A prominent difference is that in bacteria, initiator tRNA (transfer RNA) has a modified methionine, where in eukaryotes and archaea, the initiator tRNA holds an unmodified methionine.
Archaea Reproduction
Archaea reproduce by binary fission. In this process, archaeal DNA replicates, and the two DNA strands are pulled apart as the cell grows. In some cases, named multiple fission process, more than two daughter chromosomes can also be created. Unlike many bacteria and eukaryotes, archaea do not produce spores.
Archaea are also capable of unique metabolic reactions. For instance, methanogenesis, the production of methane as a metabolic by-product happens only in the archaeal domain of life. Similarly, some archaea are known to use light as a source of energy but the classical chlorophyll based photosynthesis system is absent.
Archaea and Disease
Archaea are as ubiquitous as bacteria. Despite their prevalence, no human pathogenic archaea have been found. Recently, scientists found that archaea are indeed able to colonize and survive in humans. Anaerobic archaea have been found residing in the human mouth, colon, vagina, and recently also on the skin. Being strictly anaerobic, they possibly cohabitate with anaerobic bacteria.
Anaerobic bacteria, in the human gut, help in decomposing food elements to simple hydrogen and carbon dioxide. Despite human contact with the halophiles archaea that are found on high-salt foods such as sausages, salt pork, and fish, methanogens archaea are the only ones that reside in humans. It is suggested that the job of methanoarchaea is to lower the levels of hydrogen in the gut that is produced by anaerobic bacteria. Recently, disturbance of the archaeal flora of intestine has been suggested to play an important role in triggering obesity in people.
However, the details of archeal flora competition with normal human bacterial flora are largely unavailable. Methanogens have also been found in the hindguts of arthropods such as millipedes, cockroaches, and termites. This possibly suggests that they have a role to play as gut flora even in other animals. In a study about the development of vaccines, when the lipids of archaeal origin were used as immune adjuvants, a rapid and prolonged specific immunity was noticed.
This certainly suggests that the human immune system can essentially identify them as foreign bodies, as antigens, or pathogens. This further strengthens the possibility that archaeal pathogens do exist. However, the identification of archaea in medical samples is often too difficult due to the need for complex culture conditions and cell staining techniques. The molecular identification of the organism is also challenged by an unfavorable ratio of human and bacterial DNA to the archaeal DNA.
Archaea and Evolution
The question about the origin of the eukaryotes has fascinated biologists for a long time. There are several theories but there is no definitive answer to the question of how simple cells evolved into far more complex cells. There must be a predecessor along the evolutionary road, but it simply does not exist in fossil records. Researchers believe that mitochondria might have been free-living bacteria and at some point in the past, were engulfed by another cell.
This is probably how complex cells with organelles evolved. After the discovery of archaea, their specific evolutionary link with eukaryotes was widely accepted. More recently a newly identified archaeal species named Prometheoarchaeum syntrophicum was thought to be the “missing link”, so to speak, that would shed light on how eukaryotes might have evolved. The name Prometheoarchaeum was in reference to the Greek god Prometheus who gave fire to man.
Dr. Nobu, a microbiologist in Japan, and his colleagues managed to grow Prometheoarchaeum in a lab which took more than a decade. In contrast to bacteria E. coli that divides once every 20 minutes, Prometheoarchaeum takes as long as 25 days. The project started back in 2006 when the researchers were looking for an organism in Pacific Ocean sediment that eats methane and might be used to clean up sewage.
Under the microscope, Prometheoarchaeum is a strange creature. It starts as a tiny sphere, but over the course of months, it sprouts long into branching tentacles, a kind of primitive multicellular structure. On the inside, there is no structure, just proteins, and nucleic acids. Dr. Nobu believes that during evolution archaea like Prometheoarchaeum dragged bacteria into its web of tentacles, leading to a further intimate association between archaea and the bacterial cell.
Takeaways
Like bacteria, archaea represent a large diversity of life on earth. They are important for biogeochemistry as the primary producer of methane. Evolutionarily, archaea are much closer to eukaryotes, even though morphologically similar to bacteria, calling them prokaryotes would be a misnomer.
They certainly represent a mysterious novel domain of life. The genetically distinct nature of archaea and the length of evolutionary history could hold keys to our understanding of our evolutionary past.
References
- Woese, Carl R., and George E. Fox. “Phylogenetic Structure of the Prokaryotic Domain: The Primary Kingdoms.” Proceedings of the National Academy of Sciences 74, no. 11 (November 1, 1977): 5088–90. https://doi.org/10.1073/pnas.74.11.5088.
- “Archaea.” In Wikipedia, May 16, 2020. https://en.wikipedia.org/w/index.php?title=Archaea&oldid=957054620.
- Encyclopedia Britannica. “Archaea – Characteristics of the Archaea.” Accessed May 21, 2020. https://www.britannica.com/science/archaea.
- “Archaea – Encyclopedia of Life.” Accessed May 18, 2020. https://eol.org/docs/discover/archaea.
- Bang, Corinna, and Ruth A. Schmitz. “Archaea Associated with Human Surfaces: Not to Be Underestimated.” FEMS Microbiology Reviews 39, no. 5 (September 1, 2015): 631–48. https://doi.org/10.1093/femsre/fuv010.
- Canfield, Donald E., Erik Kristensen, and Bo Thamdrup. “Systematics and Phylogeny.” In Advances in Marine Biology, edited by Donald E. Canfield, Erik Kristensen, and Bo Thamdrup, 48:1–21. Aquatic Geomicrobiology. Academic Press, 2005. https://doi.org/10.1016/S0065-2881(05)48001-3.
- “Crenarchaeota.” In Wikipedia, April 14, 2020. https://en.wikipedia.org/w/index.php?title=Crenarchaeota&oldid=950873257.
- Dodds, Walter K., and Matt R. Whiles. “Chapter 9 – Microbes and Plants.” In Freshwater Ecology (Third Edition), edited by Walter K. Dodds and Matt R. Whiles, 211–49. Aquatic Ecology. Academic Press, 2020. https://doi.org/10.1016/B978-0-12-813255-5.00009-0.
- Eckburg, Paul B., Paul W. Lepp, and David A. Relman. “Archaea and Their Potential Role in Human Disease.” Infection and Immunity 71, no. 2 (February 1, 2003): 591–96. https://doi.org/10.1128/IAI.71.2.591-596.2003.
- Eme, Laura, and W. Ford Doolittle. “Archaea.” Current Biology 25, no. 19 (October 5, 2015): R851–55. https://doi.org/10.1016/j.cub.2015.05.025.
- “Euryarchaeota.” In Wikipedia, May 9, 2020. https://en.wikipedia.org/w/index.php?title=Euryarchaeota&oldid=955742473.
- Frazer, Jennifer. “Archaea Are More Wonderful Than You Know.” Scientific American Blog Network. Accessed May 20, 2020. https://blogs.scientificamerican.com/artful-amoeba/archaea-are-more-wonderful-than-you-know/.
- “Introduction to the Archaea.” Accessed May 18, 2020. https://ucmp.berkeley.edu/archaea/archaea.html.
- “Isolation of an Archaeon at the Prokaryote–Eukaryote Interface.” Accessed May 21, 2020. https://www.ncbi.nlm.nih.gov/pmc/articles/PMC7015854/.
- “Last Universal Common Ancestor.” In Wikipedia, May 7, 2020. https://en.wikipedia.org/w/index.php?title=Last_universal_common_ancestor&oldid=955308140.
- Schopf, J. William. “Fossil Evidence of Archaean Life.” Philosophical Transactions of the Royal Society B: Biological Sciences 361, no. 1470 (June 29, 2006): 869–85. https://doi.org/10.1098/rstb.2006.1834.
- “This Strange Microbe May Mark One of Life’s Great Leaps – The New York Times.” Accessed May 21, 2020. https://www.nytimes.com/2020/01/15/science/cells-eukaryotes-archaea.html.