Cells are the fundamental unit of life, the basic building blocks that make up all living things. From the microscopic amoebas to the mammoth blue whale, these cells contain all the essential machinery for maintaining life. Every cell is surrounded by a semi-permeable layer called the cell membrane.
The cell membrane is a protective boundary that separates the constituents of the cell from its outside (extracellular space). Enclosed within this membrane lies a solution called the ‘cytoplasm’ in which all the cell’s constituents are suspended. Cell membranes are typically thin, ranging between 4-12 nanometers. However, some specialized cells can contain thicker boundaries.
The cell membrane doesn’t just function as a boundary, it also transports molecules across the membrane, regulates adhesion to surfaces and transmits signals from one cell to another.
To perform these divergent functions, the cell membrane is equipped with a unique combination of complex proteins, lipids and carbohydrates. In this article, we explore the structures, composition, diversity and functions of cell membranes in living things.
Discovery of the Cell Membrane
The invention of the microscope in the 17th century enabled the magnification and visualization of cells. In 1665, Robert Hooke isolated dead cells from a cork (a type of plant) and described its structure to be honey-comb shaped, enclosed by thick walls. Although animal cells proved harder to isolate and magnify, it was theorized at the time that they would contain the same hard boundaries as plant cells.
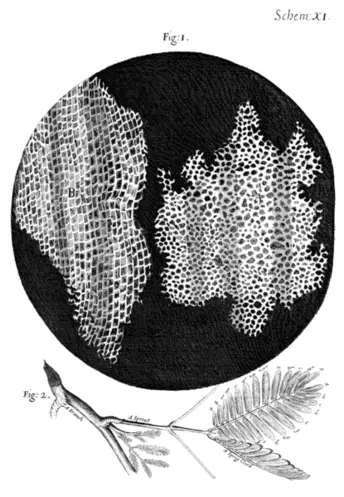
However, in the 19th century, observational experiments demonstrated that animal cells were more permeable and flexible compared to plant cells. In 1877, the botanist Wilhelm Pfeffer proposed the membrane theory which postulated that animal cells were enclosed by a very thin, flexible barrier that allowed some molecules to pass through.
Around the same time, Georg Quincke also noted that animal cells when dropped in water, formed spherical shapes that split into two halves when broken, quite similar to how an oil droplet behaves. Ernest Overton speculated that the cell membrane was made of lipids based on its solubility in fat-based solutions.
The ability of lipid molecules to slide past each other allowed animal cells to be more permeable and flexible than their plant counterparts. However, the consensus at the time was that cell membranes were a secondary structure that was not important to the function of the cell.
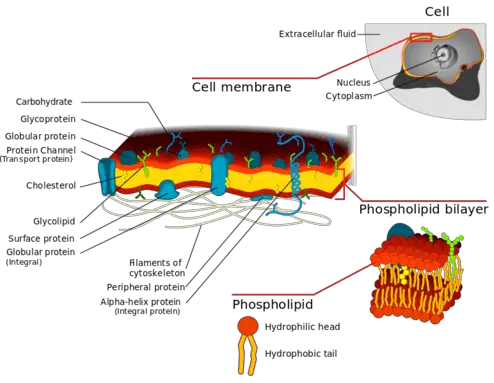
In 1925, Gorter and Grendel pioneered the elucidation of the bilayer structure of cell membranes using crystallization experiments. They speculated that animal cell membranes were made up of two layers of lipids arranged concurrently against each other. The thickness of these layers were determined to be 3-4 nanometers based on light refraction experiments.
In 1935, it was observed that the surface tension of cell membranes was lower than what it was expected to be based on the calculated surface tension of lipids. This finding gave rise to the theory that protein layers were enclosed between the lipid layers, thus lowering the interfacial surface tension. This model, called the paucimolecular model was widely accepted by experts until Singer and Nicolson proposed the fluid mosaic model in 1972. The fluid-mosaic model proposed that proteins in a cell membrane float in a lipid bilayer “sea”.
Although this is a simplistic visualization of the real thing, the fluid-mosaic model is still widely accepted today. Further, modern advances in microscopy and structural studies have allowed the elucidation of the complexity of the cell membrane.
The Phospholipid Bilayer
The cell membrane primarily consists of a phospholipid bilayer. These lipids can be divided into two distinct regions; a hydrophobic (water-fearing) tail and a hydrophilic (water-soluble) head. The cell membrane spontaneously arranges to hide the hydrophobic tails inside with the heads facing the surrounding water in the extracellular and intracellular region.
This results in a continuous bilipid layer wherein the hydrophobic tails prevent the polar molecules inside the cell (DNA, proteins, carbohydrates) from leaking outside. In some regions, the cell membrane is interspersed with specialized protein complexes that carry out transport and communication.
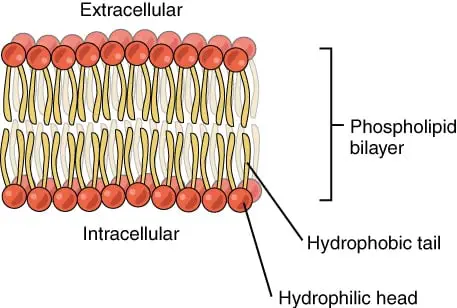
It should be noted the cell membrane is not an immobile structure. It is constantly moving depending on the molecules being transported across. Imagine putting your hand into a clump of flexible clay. The material will temporarily part to let your finger pass through, but once you remove your hand, the clay falls back into place. Therefore, the structures we describe below are merely snapshots of a time in the cell.
Composition of the Cell Membrane
The cell membrane is called a ‘mosaic’ because it is composed of a variety of components such as lipids, proteins and carbohydrates. Just like its structure, the composition of a cell membrane is not constant and can vary according to the function being performed. For example, the amount of cholesterol in neurons can change according to the stage of development. Here we the most common molecules found in the cell membrane.
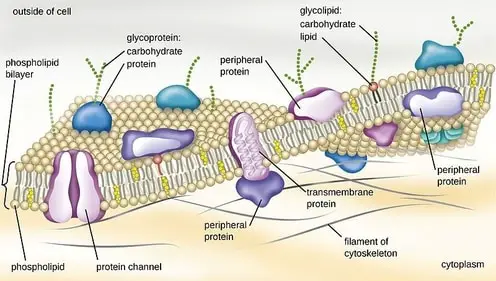
Lipids
Cell membranes of animals primarily contain three classes of lipids namely phospholipids, glycolipids and sterols. The proportion of these components can vary from cell to cell, or even depend on external conditions. However, across all cell membranes, phospholipids are the most abundant, constituting nearly 50% of the membrane structure.
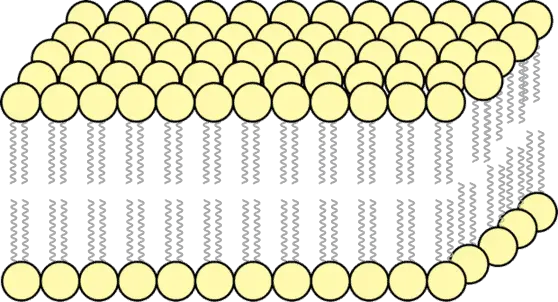
Phospholipids as previously described, contain two long chains of fatty acids that are attached to a polar head. The tails which are primarily built with fatty acids repel water. On the other hand, the head is attached to a phosphoryl group which can dissolve in water. Therefore the phospholipid is amphiphilic – meaning they are both hydrophobic and hydrophilic. When added to an aqueous solution, phospholipids spontaneously rearrange into an energetically favorable state with the tails tucked inside and the heads facing outside.
The composition of sterols and glycolipids can profoundly affect the fluidity of the membrane. Sterols and glycolipids are structurally different from the phospholipids which build the membrane. This creates a kink in the structure preventing the fatty acids from being packed together, thus increasing the porosity and fluidity of the membrane. The phenomena is referred to as homeoviscous adaptation.
Cholesterol is generally found in small quantities, interspersed between the hydrophobic tails of phospholipids. The amount of cholesterol found in the membrane can depend on the cell type or organism type, with the amount of cholesterol being proportional to the stiffness and strength of a membrane. In high-temperature conditions, cholesterol reduces the fluidity and thus permeability of a membrane with the opposite being true in colder conditions.
Cholesterol production can also be triggered by colder temperatures, thus cold-weather animals seem to contain more cholesterol and thicker cell membranes than their warm-weather counterparts.
Proteins
Proteins are a major component of the cell membrane coming only second to phospholipids. Although proteins can regulate the fluidity of the membrane, they primarily function as specialized machines for transport or communication. Depending on their position within the membrane these can be integral or peripheral.
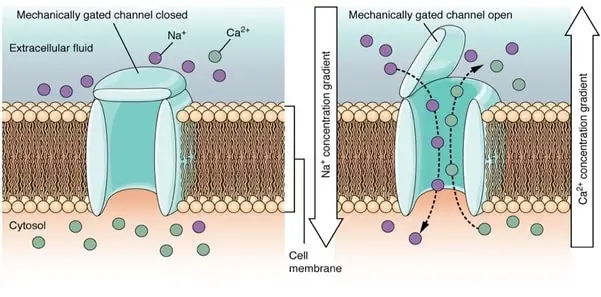
Integral membrane proteins are found integrated into the hydrophobic regions of the cell membrane. As a result, these proteins have at least one hydrophobic portion which interacts with the tails of phospholipids.
While some integral membrane proteins are only partially immersed into the membrane some may extend all the way across (transmembrane proteins). Some transmembrane proteins like the G-protein coupled receptor protein can pass through the membrane over 12 times! Other examples of integral proteins include ion “gates” which allow specific ions to pass through.
Peripheral membrane proteins are generally found on the surface of membranes. However, some may be bound to integral or lipid-anchored proteins thus forming a complex.
Unlike integral proteins, these do not have hydrophobic regions and can therefore not be immersed into the phospholipid tails. Some peripheral proteins only transiently attach to cell surfaces for performing specific functions.
Carbohydrates
Carbohydrates can be found on the surface of cell membranes or bound to proteins and lipids. Generally, these carbohydrates are small, with 20-60 monosaccharide units which can be linear or branched. The most common carbohydrate found in animal cells include glycoproteins such as cerebrosides and gangliosides.
These molecules are primarily involved in cell-cell communication, with every cell having a distinct composition of carbohydrates in the form of a ‘blueprint’. Carbohydrates are particularly important in the cells of the immune system where it recognizes, binds and attacks invading pathogens. In some cells, glycocalyx carbohydrates also assist the cell in adhering to a surface and moving from one place to another.
Functions of a Cell Membrane
Cell membranes perform a diverse range of functions. Primarily, they regulate the exit and entry of materials from the cell. Some of these materials may be required for metabolism or other biological functions in the cell. Further, by regulating molecular exchanges, cell membranes can also control the polarity, pH and osmotic pressure of a cell. Some transport mechanisms used by the cell membrane are summarized here.
Diffusion or Passive transport: Small molecules such as carbon dioxide (which is a waste product of metabolism) or oxygen (which is essential for producing energy) can passively diffuse across the membrane, moving from a region of high concentration to a region of low concentration. It is called “passive” because the cell does not have to utilize energy to facilitate the movement. In biological systems, movement of water molecules across a concentration gradient is termed ‘osmosis’.
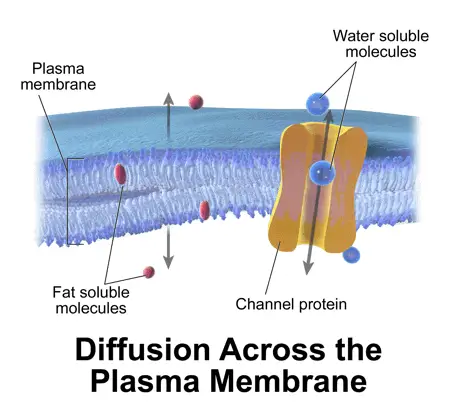
Transporters/ Channels or Gates: Large molecules, particularly polar molecules, cannot move through the hydrophobic phospholipid layer. In such cases, movement is facilitated by a set of specialized protein complexes called transmembrane proteins. These proteins have functional sides facing both extracellular and intra-cellular regions that regulate the movement of sugars or amino acids.
Transporters are classified, according to the type of molecule they transport. For example, aquaporins allow small molecules to passively transport across them, while transmembrane transporters actively pump molecules through them using cellular energy. Protein channels such as permeases are highly specific. They recognize certain molecules by shape and only allow those to pass through.
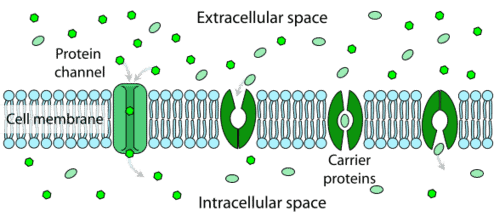
Endocytosis: Endocytosis refers to the process by which cells absorb molecules by engulfing them. The cell membrane folds inwards forming a small deformation that is called an ‘invagination’. The molecule to be moved into the cell is captured within the invagination.
These movements are facilitated by a set of specialized proteins present on the cell membrane. After the molecule is engulfed, the proteins pinch off the invagination, forming a vesicle around the captured substance. The vesicle can then be used to transport the molecule to any part of the cell.
Phagocytosis refers to the process of engulfing and destroying molecules such as bacteria or metabolic wastes. On the other hand, the capture of small ions and molecules important to the function of a cell is referred to as pinocytosis. Endocytosis is a form of active transport and requires the use of cellular energy.
Exocytosis: Exocytosis is just the opposite of endocytosis. Here the vesicle moves to the edge of a cell membrane and fuses with it, thus creating a protruding deformation that extends into the extracellular space. Exocytosis is used to remove substances that cannot be degraded by the cell. These could include by-products of metabolism and other toxins. Furthermore, hormones and enzymes which need to be secreted into the bloodstream but cannot pass through the plasma membrane can also be moved through exocytosis.
The first step of exocytosis involves the formation of the vesicle which contains the substance to be transported. This is carried out by a specialized factory located inside the cell called the Golgi apparatus. The cytoskeleton filaments, which are a complex set of underlying proteins that function as the cells’ limbs, move the vesicle from the center of the cell to the cell membrane. Subsequently, the membrane of the vesicle fuses with the cell membrane. A small passage is formed across the fused membrane by rearrangement of lipids, through which the molecule is transported.
Cell membranes also function as signaling machines which transmit signals from one cell to another. They are major actors in the response of the cell to external stimuli. Furthermore, cell membranes are hydrophobic and therefore accumulate lipid pigments and hydrophobic proteins within the cell which bind to their specific receptors and stimulate metabolic or bioenergetic activities.
Cell signaling occurs through specialized peripheral proteins integrated into the cell membrane. These signaling proteins can be peripheral or integrated proteins depending on their location in the cell membrane. These proteins or protein complexes are called receptors because they receive signals from the surrounding environment and transmit it into the cell. A specific signal or “ligand” binds to a highly specialized receptor which sets off a cascade of signals that ultimately result in a response.
For example, binding of insulin to the insulin receptor in the muscle and fat cells sets off a set of insulin signaling events which moves glucose from the bloodstream into the cells.
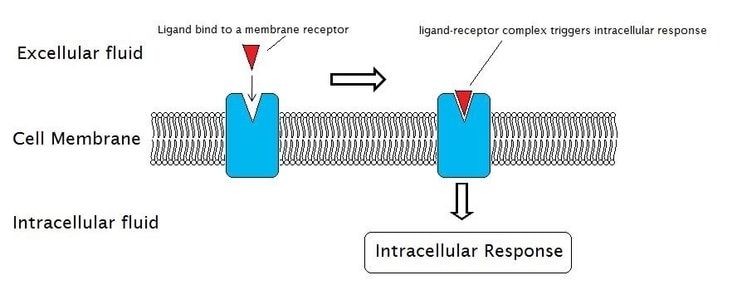
The cytoskeleton refers to a set of complex molecules that underlie the cell. These proteins working together act as the limbs of the cell, adhering the cell to the surface and helping move molecules across the cell.
The cell membrane plays an important role in anchoring the cell to the cytoskeleton thus providing a specified shape to the cell. The cell membrane via the cytoskeleton also attaches to the extra-cellular matrix and neighboring cells in order to form tissues.
Specialized Cell Membranes
The nucleus is the epicenter of a cell, containing the instructions for all processes essential to life. In order to protect the nucleus, these structures are generally bound by a well-defined nuclear membrane. Prokaryotes are primitive organisms which do not contain a well-defined nucleus or other cell organelles.
These organisms contain cell membranes that are structurally distinct from others. Furthermore, some prokaryotes have specialized cell membranes that have evolved to perform specific functions. A few examples are described below.
Gram Negative bacteria contain a cell membrane that is surrounded by another outer membrane. A solution called periplasm lies in between the two. The outer membrane differs from most other organisms because it is made of phospholipids facing the exterior space with lipoproteins packed inside (the opposite of other phospholipid membranes).
Unlike the cell membrane, the outer membrane is quite porous due to the presence of pore-forming proteins. It is believed that the outer membrane evolved in order to allow the microorganisms to adapt to various environmental conditions. For example, under room temperature, the bacteria Staphylococcus aureus appears to be gel-like. However, when the temperature drops, the outer membrane hardens, thus protecting its contents.
Some bacteria have cell membranes containing specialized lipopolysaccharides which are a combination of complex carbohydrates and lipids. These molecules play a role in the bacteria’s defenses. When the organisms encounter an attack from microorganisms or environmental stress, the proteins cause the plasma membrane to protrude outside, forming a vesicle which contains all the necessary components for fighting against the attack.
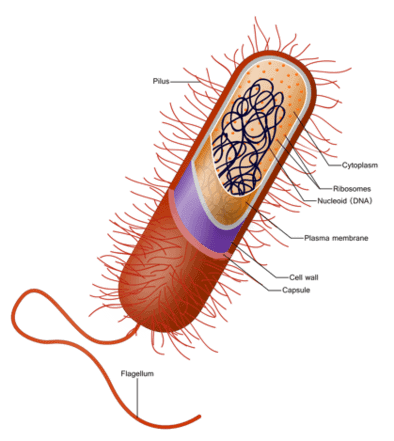
Eukaryotes refer to organism which contain cells with well-defined nucleus. Some Eukaryotic cells have evolved different types of membranes in order to perform specialized functions. Cells in the muscle have to be able to contract and expand when they receive a signal from the brain.
They contain specialized cell membranes called sarcolemma. Sarcolemma have distinct channels that stretch from one end of a muscle cell to another, through which signals are transmitted from one cell to another. Furthermore, sarcolemma is considerably thicker than other cell membranes, reaching up to 10 nanometers in thickness.
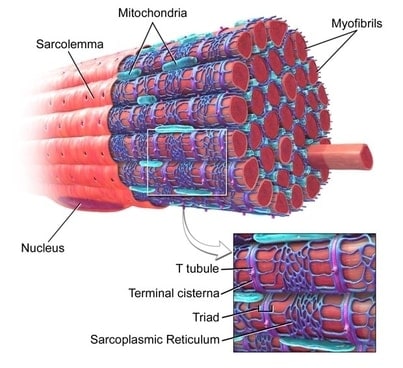
The eggs found in the ovary are called oocytes. In order to be fertilized, these eggs need to be penetrated by a sperm. Cell membranes of oocytes, called oolemma are specialized for this purpose.
These membranes do not contain a bilayer of phospholipids. Instead, oocytes are made up of two layers; an exterior boundary of glycoproteins called the corona radiata, and an inner envelope called the zona pellucida. The sperm must invade both the layers in order to fertilize the cell.
The cells of the brain, called neurons, are some of the most specialized signal transmitters on earth. They contain a head called the cell body which is attached to a long tail that is called the axon.
The cell membranes of neurons are adapted to transmit a signal from one neuron to the next. They contain densely packed lipid bilayers that are firmly attached to the underlying cytoskeleton structures.
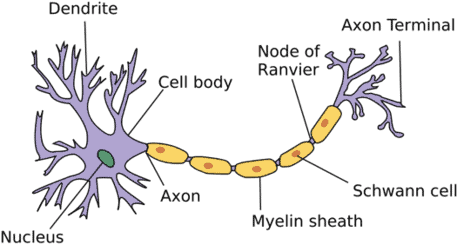
How Viruses Attack Cell Membranes
Viruses are some of the most dangerous parasites on the planet, despite their tiny size. They can hijack the cell’s machinery and use it to make multiple copies of themselves which can then be released to go infect other cells. A decade of research has revealed the precise mechanisms by which a virus manages to evade the protective mechanisms of the cell membrane in order to enter the cell.
An single unit of the virus, called the virion is a very primitive structure that contains genetic material surrounded by a protein envelope. In fact, it is so primitive that scientists debate if a virus is truly “alive”. Some viruses may also contain an outer membrane that surrounds the envelope. Interestingly, this outer membrane is built using their host organisms cell materials!
When a virus encounters a cell, its outer membrane may directly fuse with the cell membrane of the host organism. This fusion is enabled by a set of fusion proteins present on the viral envelope. The virus scans the surface of the host cell, looking for host receptors that can be attacked by the fusion protein. Once the fusion protein is activated, two monolayers of the viral and host cell membrane touch each other by a process called hemifusion. Subsequently, the leftover monolayers of the bi-layered membrane collapse to form a hemifusion diaphragm.
In healthy cells, this diaphragm prevents the viral genome from infecting the cell. However, some fusion proteins can attack this diaphragm to create a pore through which the viral genome can enter the cell.
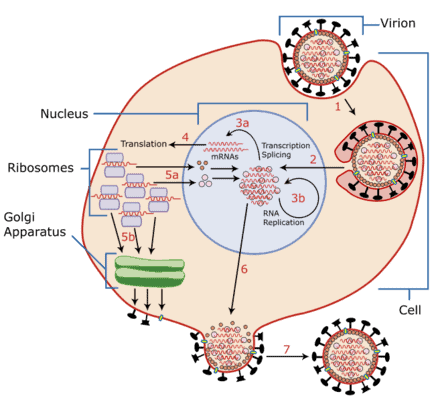
On the other hand, some viruses are engulfed and absorbed into the cell by endocytosis. Subsequently, the virus attacks an organelle inside the cell, such as the Golgi apparatus, following which infection occurs. Once a new virion is created inside the cell, it buds out, fusing with the host cell’s membrane. In this way, the virion captures the host cell membrane to form its outer envelope.
These virions which contain an outer membrane are called enveloped viruses. Scientists who are trying to prevent viral attacks are trying to understand how the fusion of the viral envelope with the host cell’s membrane can be prevented. Some vaccines against the influenza virus have been developed which can block the action of viral fusion proteins.
Takeaways
The cell is the body’s principal soldier, carrying out all the functions that are essential to life. The development of the cell membrane that protects this soldier from the environment was likely one of the most important steps in the evolution of life.
Yet, cell membrane biology is not a completed quest. The structure of this complex boundary has not been fully elucidated but every day, scientists are delving further into deciphering the functions of membrane components.
References
- Cohen FS. How Viruses Invade Cells. Biophys J. 2016;110(5):1028-1032. doi:10.1016/j.bpj.2016.02.006
- Davson H: Growth of the concept of the paucimolecular membrane. Circulation. 1962, 26: 1022-1037. 10.1161/01.cir.26.5.1022
- Edidin M: Lipids on the frontier: a century of cell-membrane bilayers. Nat Rev Mol Cell Biol. 2003, 4: 414-418. 10.1038/nrm1102
- Kleinzeller A: The postulate of the cell membrane. A history of biochemistry Exploring the cell membrane: conceptual developments. 1995, Elsevier Science, Amsterdam, 27-90. eBook ISBN: 9780444598196
- Kozlov M.M., Campelo F., McMahon H.T. Mechanisms shaping cell membranes. Curr. Opin. Cell Biol. 2014;29:53–60. 10.1016/j.ceb.2014.03.006
- Lombard, J. Once upon a time the cell membranes: 175 years of cell boundary research. Biol Direct 9, 32 (2014). https://doi.org/10.1186/s13062-014-0032-7
- Robertson JD: Membrane structure. J Cell Biol. 1981, 91: 189-204 10.1083/jcb.91.3.189s
- Singer SJ, Nicolson GL: The fluid mosaic model of the structure of cell membranes. Science. 1972, 175: 720-731. 10.1126/science.175.4023.720
- Tanford C: The Hydrophobic Effect. Formation of Micelles and Biological Membranes. 1973, Wiley, New York https://doi.org/10.1021/ed058pA246.1