Cell organelles, like organs within the human body, each perform a specific function within the cell. Cells are the building blocks of all life on the planet and without cells humans along with millions of other species would not exist. There are many important organelles within a cell but one in particular is known as the “powerhouse” and for good reason.
Mitochondria is an extremely important organelle found in most eukaryotic cells and is responsible for the production of Adenosine Triphosphate (ATP) which is a compound responsible for providing energy the fuels many of the processes of the cell.
In this post we will take a closer look at the structure, function, and role in the innerworkings of a cell and its metabolism.
Mitochondria Strcture
Mitochondria shape and size is highly variable. Each mitochondrion has a limiting outer membrane that surrounds the organelle, an intermembrane space that separates the outer and inner membrane. It also has a matrix (similar to the cytoplasm but more viscous) that is enclosed by the inner membrane, and its own circular DNA and ribosomes in the matrix (figure 1).
The inner membrane form folds called cristae that help increase the surface area where reactions occur since the inner membrane is the principal site of energy production. Through the cristae, the F1-F0 ATP synthase protein produces the majority of the ATP used by the cells. The mitochondrial matrix is the site of mitochondrial DNA replication, transcription, protein biosynthesis and other enzymatic reactions (Munn 1974, Rogers 2010, Scheffler 2011, Kühlbrandt 2015, Know 2018).
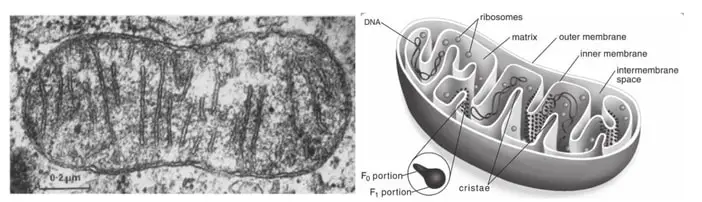
Mitochondria were essential for the development of life as we know it due to their multiple functions, especially ATP production. Additionally, they are also involved in the generation and detoxification of reactive oxygen species, apoptosis, cellular differentiation, intracellular calcium regulation, urea cycle, lipid metabolism and interconversion of amino acids, among others (Rogers 2010, Scheffler 2011).
Brief History
In 1850, reports of structures inside cells with similar characteristics to those of mitochondria (described as grains) start to appear. In 1888, after some improvements in staining, more proper morphological descriptions were made, including observations made by Albert von Köllinker when micro-dissecting these grains from insect muscle cells that reached the conclusion that mitochondrion have a membrane.
Later, in 1898, Carl Benda named them from the Greek [mithos] ‘thread’ and [chondros] ‘granules’. Kingsbury in 1912 and Lehninger and Warburg in 1913, found that mitochondria were associated with respiration, but it took another 40 years of biochemical research to identify the mitochondria as the “powerhouse of the cell” (Scheffler 2011).
Mitochondria and Chloroplast
First, some background. A major point in the evolution of life as we know it was the development of prokaryotes capable of photosynthesizing and producing molecular oxygen. These organisms allowed the atmosphere to become rich in oxygen and promoted the evolution of other prokaryotes to more efficient aerobic forms of metabolism approximately 2 billion years ago.
The most accepted theory stated that eukaryotic cells became aerobic, engulfing and developing a symbiotic relationship with aerobic prokaryotes that evolved into mitochondria. Eukaryotic cells containing mitochondria then developed an endosymbiotic relationship with photosynthetic prokaryotes that evolved into chloroplasts.
Comparatively speaking, both mitochondria and chloroplast have a similar origin, the have outer and inner membranes, transform energy into a form that the cell can use, and have their own DNA and protein-synthesizing machinery. But, unlike mitochondria, chloroplasts are only found in plants, contain different structures (like thylakoids and stroma), pump protons across the membrane using the sun’s energy, and produce oxygen (Rogers 2010, Known 2018). Putting this in perspective, mitochondria seem to be more distant cousins than twins.
Mitochondria and Cellular Respiration
The metabolic processes that allow the cell to get energy from food are known as cellular respiration. Many of these processes occur in mitochondria and utilize the energy from sugars, carbohydrates, fats and proteins to generate adenosine triphosphate, commonly known as ATP. Cellular respiration has three main steps for aerobic respiration (the most common process in plants and animals, requires oxygen to produce energy).
- Glycolysis.
- The citric acid cycle.
- The electron transport chain (Disha Experts 2020).
Glycolysis is the first step and is considered anaerobic because doesn’t require oxygen to happen. In essence, this process is characterized by the breakdown of glucose in half, producing two molecules of pyruvic acid. This process occurs in the cell’s cytoplasm and has a net gain of 2 ATP (requires two molecules of ATP and produces four molecules of ATP) (Patton 2015, Disha Experts 2020).
The pyruvate acid molecules produced by glycolysis are then transported to the mitochondria. Once in the intermembrane space, the enzyme pyruvate dehydrogenase is responsible to form Acetyl CoA and the next step of the aerobic cellular respiration starts (figure 2).
The tricarboxylic acid cycle, also known as the citric acid cycle or the Krebs cycle is the major pathway responsible for producing energy. Here, a series of reactions occur where every product of the reaction becomes the substrate needed for the following reaction. The majority of the energy released by this process is due to the electrons (e–) made by the oxidative reactions and their accompanying protons (H+), transferred to NAD+ to produce NADH and FAD to produce FADH2.
As a result, 12 ATP are produced for each molecule of acetyl CoA that enters the cycle, because two molecules of acetyl CoA are formed from one molecule of glucose, the total energy production of the cycle is 24 ATP (Patton 2015, Demirel & Gerbaud 2019, Disha Experts 2020). As shown in figure 3, NADH transfer electrons to a protein complex (complex I) embedded in the cristae of the mitochondria, and became the first step to the creation of a proton gradient that drives ATP production by the ATP synthase.
The electrons from the oxidation of NADH are transferred to a quinol, pumping four protons from the matrix to the cristae, then protein complex II transfer the electrons from the reduced quinol to cytochrome c, pumping one proton to the cristae, and finally, protein complex IV transfer the electrons from cytochrome c to molecular oxygen, pumping four protons for each oxygen molecule.
Protein complex II doesn’t contribute to the electron gradient but transfers electrons from succinate to quinol. Ultimately, protein complex V, better known as F1-F0 ATP synthase, uses the proton gradient to produce ATP, transferring protons from the cristae to the matrix (Patton 2015, Kühlbrandt 2015, Demirel & Gerbaud 2019, Disha Experts 2020).
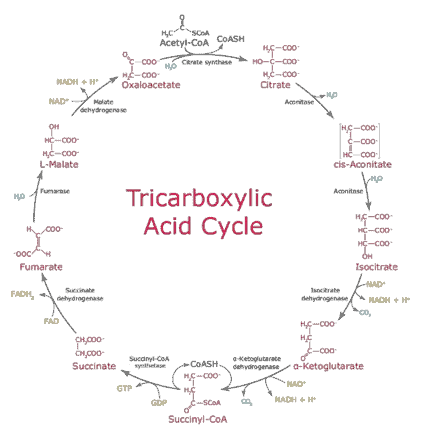
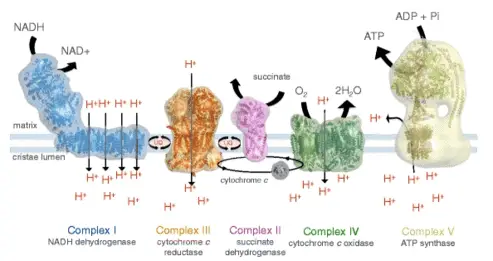
Reactive Oxygen Species
Reactive oxygen species (ROS) are chemically reactive species that contain oxygen. In mitochondria, ROS are generally produced in the electron transport chain, due to the reduction of oxygen by single electrons from protein complex I and III, which lead to the formation of superoxide and subsequently to hydrogen peroxide.
Low levels of ROS can be a positive regulatory signal, but high levels result in tissue aging due to decreased metabolic function and energy production, a consequence of significant damage to cell structures, capable of inducing cell death (Chial & Craig 2008, Scheffler 2011).
Mitochondrial DNA
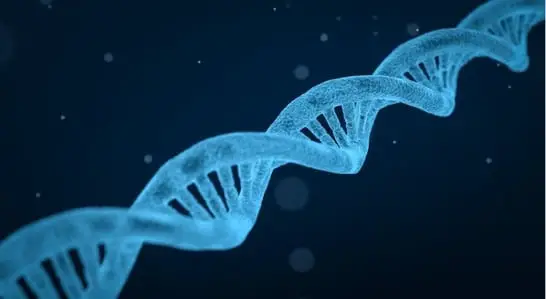
Mitochondrial DNA in humans is always inherited from the mother, this means that we share our mitochondrial DNA sequence with our maternal relatives. Despite the high mutation rates and variability that exist in mitochondrial DNA sequences, maternally related individuals like a grandmother and her grandson can be easily matched.
This is essential to identify individuals separated from their families and has been extremely important in human rights cases. Dr. Mary-Claire King is an outstanding researcher that worked on human rights issues and used mitochondrial DNA sequences as a tool to establish relationships with maternal relatives.
One remarkable case occurred after the military dictatorship in Argentina where between 9000 and 30000 people were kidnapped, including 220 infants and children. In 1977, the grandmothers of these children constitute an association to identify their missing grandsons and granddaughters, some being illegally adopted by military families.
In 1984, more than 60 orphans were matched with their biological families using mitochondrial DNA sequence data. To make this happen, Dr. King collected blood samples of orphan children and women who had lost their relatives (Chial & Craig 2008).
Mitochondria and Diseases
Mitochondrial diseases are disorders of the mitochondrial respiratory chain due to mutations in nuclear DNA and mitochondrial DNA (mtDNA). Mutations in mtDNA have been related to severe genetic diseases in humans. These diseases seem to result from defective mtDNA, a consequence of point mutations (mutations affecting one or few nucleotides), specifically substitutions (exchange a nucleotide for another) and deletions (remove one or more nucleotides), co-occur with normal mtDNA (Ladoukakis & Zouros 2017).
Mitochondrial diseases have a wide range of symptoms and include neuropathies, myopathies, cardiovascular disorders and gastrointestinal, endocrine and hematological alterations. Some diseases related to mitochondrial mutations are diabetes, Huntington’s disease, cancer, Alzheimer’s, Parkinson, deafness, cardiovascular disease, sarcopenia, chronic fatigue syndrome (figure 4) (Gorman 2016, Suárez-Rivero et al. 2017).
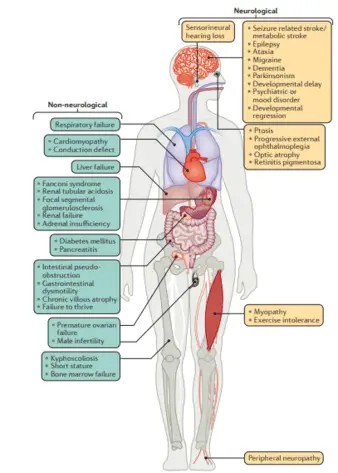
The number of mitochondria per cell is highly variable and ranges from hundreds to thousands and varies from one cell type to another, due to this there is more mitochondrial DNA than nuclear DNA inside a cell.
Takeaways
In summary, mitochondria are super powerful and small organelles inside eukaryotic cells that allow us to extract energy from the oxygen we breathe and the food we eat.
Additionally, they have their own machinery to synthesize proteins and their own DNA, which has allowed us to identify family ties thanks to the mitochondrial DNA sequences that we share with our maternal relatives.
But with great power comes great responsibilities, and damage or dysfunction of mitochondria generates severe problems, including cell damage, cell death and mitochondrial diseases. Certainly, an amazing organelle to review.
References
- Chial, H. & Craig, J. (2008) mtDNA and mitochondrial diseases. Nature Education 1(1):217
- Demirel, Ya. & Gerbaud, V. (2019). “Thermodynamics and Biological Systems” in Nonequilibrium Thermodynamics: Transport and Rate Processes in Physical, Chemical and Biological Systems. Fourth Edition. Transport and Rate Processes in Physical, Chemical and Biological Systems, Chapter 11, pp. 489-571.
- Disha Experts. (2020). NEET 2020 Biology Guide. 7th edition. Disha Publications. 856 pp.
- Gorman, G. S., Chinnery, P. F., DiMauro, S., Hirano, M., Koga, Y., McFarland, R., … & Turnbull, D. M. (2016). Mitochondrial diseases. Nature reviews Disease primers, 2(1), 1-22.
- Kühlbrandt, W. (2015). Structure and function of mitochondrial membrane protein complexes. BMC biology, 13(1), 89.
- Ladoukakis, E. D., & Zouros, E. (2017). Evolution and inheritance of animal mitochondrial DNA: rules and exceptions. Journal of Biological Research-Thessaloniki, 24(1), 2.
- Know, L. (2018). Mitochondria and the Future of Medicine: The Key to Understanding Disease. Chronic Illness, Aging, and Life Itself. Chelsea Green Publishing Co.
- Munn, E. A. (2014). The structure of mitochondria. Academic Press. 480 pp.
- Patton, K. T. (2015). Anatomy & Physiology-E-Book. Elsevier Health Sciences. 1264 pp.
- Rogers, K. (Ed.). (2010). The cell. Britannica Educational Publishing. 240 pp.
- Scheffler, I. E. (2011). Mitochondria. John Wiley & Sons. 480 pp.
- Sigma Aldrich BioFiles. (2007). The Krebs cycle — harnessing chemical energy for cellular respiration. BioFiles, 2.6, 16.
- Suárez-Rivero, J. M., Villanueva-Paz, M., la Cruz-Ojeda, D., De la Mata, M., Cotán, D., Oropesa-Ávila, M., … & Sánchez-Alcázar, J. A. (2017). Mitochondrial dynamics in mitochondrial diseases. Diseases, 5(1), 1.